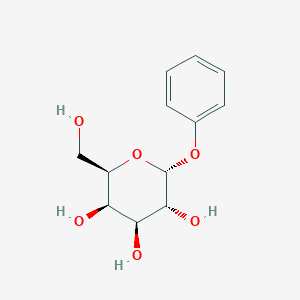
Phenyl-alpha-D-galactopyranoside
Overview
Description
Phenyl-alpha-D-galactopyranoside is a substituted galactoside, a type of carbohydrate derivative It consists of a galactose molecule bonded to a phenyl group
Mechanism of Action
Target of Action
Phenyl-alpha-D-galactopyranoside primarily targets alpha-glucosidase , an enzyme that plays a critical role in the breakdown of carbohydrates into simple sugars. This enzyme is considered a key target in the prevention and treatment of type 2 diabetes .
Mode of Action
This compound interacts with its target, alpha-glucosidase, by acting as a substrate . The compound is hydrolyzed by the enzyme, leading to the release of phenol . This interaction can be detected through a colorimetric assay, enabling the quantification of alpha-glucosidase activity .
Biochemical Pathways
The hydrolysis of this compound by alpha-glucosidase is part of the broader carbohydrate metabolism pathway . This process is crucial for the breakdown of complex carbohydrates into simple sugars, which can then be absorbed and used for energy production .
Pharmacokinetics
As a substrate for alpha-glucosidase, it is likely to be metabolized in the gastrointestinal tract where this enzyme is found
Result of Action
The hydrolysis of this compound by alpha-glucosidase results in the release of phenol . This reaction can be used to measure the activity of alpha-glucosidase, providing a useful tool for studying carbohydrate metabolism and related disorders, such as diabetes .
Action Environment
The action of this compound is influenced by the environment in which it is present. For instance, the activity of alpha-glucosidase, the enzyme that metabolizes the compound, can be affected by various factors such as pH and the presence of other molecules
Biochemical Analysis
Biochemical Properties
Phenyl-alpha-D-galactopyranoside plays a significant role in biochemical reactions. It acts as a substrate for enzymes such as beta-galactosidase and alpha-galactosidase . These enzymes are responsible for the hydrolysis of this compound, a process that involves breaking down the compound into simpler molecules . The nature of these interactions is primarily enzymatic, with this compound serving as a key reactant in the enzymatic processes .
Cellular Effects
The effects of this compound on cells and cellular processes are largely related to its role as a substrate for galactosidases . By participating in enzymatic reactions, this compound influences cell function, including impacts on cell signaling pathways, gene expression, and cellular metabolism .
Molecular Mechanism
The molecular mechanism of action of this compound involves its interaction with enzymes such as beta-galactosidase and alpha-galactosidase . These enzymes bind to this compound, leading to its hydrolysis . This process can result in changes in gene expression and influence various cellular functions .
Metabolic Pathways
This compound is involved in metabolic pathways related to the activity of galactosidases . These enzymes interact with this compound, potentially affecting metabolic flux or metabolite levels .
Transport and Distribution
The transport and distribution of this compound within cells and tissues are likely related to its role as a substrate for galactosidases
Preparation Methods
Synthetic Routes and Reaction Conditions: Phenyl-alpha-D-galactopyranoside can be synthesized through the glycosylation of galactose with phenol. The reaction typically involves the use of a glycosyl donor, such as a galactosyl bromide, and a glycosyl acceptor, such as phenol, in the presence of a catalyst like silver carbonate. The reaction is carried out under anhydrous conditions to prevent hydrolysis of the glycosidic bond.
Industrial Production Methods: In an industrial setting, the synthesis of this compound may involve the use of enzymatic methods. Enzymes like galactosidases can catalyze the transfer of galactose from a donor molecule to phenol, producing this compound with high specificity and yield.
Chemical Reactions Analysis
Types of Reactions: Phenyl-alpha-D-galactopyranoside can undergo various chemical reactions, including:
Hydrolysis: The glycosidic bond can be hydrolyzed by acids or enzymes, yielding galactose and phenol.
Oxidation: The phenyl group can be oxidized to form phenolic derivatives.
Substitution: The hydroxyl groups on the galactose moiety can participate in substitution reactions, forming esters or ethers.
Common Reagents and Conditions:
Hydrolysis: Acidic conditions (e.g., hydrochloric acid) or enzymatic catalysis (e.g., galactosidase).
Oxidation: Oxidizing agents like potassium permanganate or hydrogen peroxide.
Substitution: Reagents like acyl chlorides or alkyl halides in the presence of a base.
Major Products:
Hydrolysis: Galactose and phenol.
Oxidation: Phenolic derivatives.
Substitution: Ester or ether derivatives of this compound.
Scientific Research Applications
Phenyl-alpha-D-galactopyranoside has several applications in scientific research:
Chemistry: Used as a model compound to study glycosidic bond formation and cleavage.
Biology: Employed in enzyme assays to measure the activity of galactosidases.
Medicine: Investigated for its potential role in drug delivery systems due to its ability to form stable glycosidic bonds.
Industry: Utilized in the synthesis of complex carbohydrates and glycosides for various industrial applications.
Comparison with Similar Compounds
Phenyl-alpha-D-galactopyranoside can be compared with other similar compounds, such as phenyl-beta-D-galactopyranoside and phenyl-alpha-D-glucopyranoside. While these compounds share structural similarities, their interactions with enzymes and their chemical reactivity can differ significantly. For instance:
Phenyl-beta-D-galactopyranoside: Has a different glycosidic linkage (beta instead of alpha), which affects its enzymatic hydrolysis.
Phenyl-alpha-D-glucopyranoside: Contains glucose instead of galactose, leading to different reactivity and biological interactions.
These differences highlight the unique properties of this compound and its specific applications in various fields.
Properties
IUPAC Name |
(2R,3R,4S,5R,6R)-2-(hydroxymethyl)-6-phenoxyoxane-3,4,5-triol | |
---|---|---|
Details | Computed by LexiChem 2.6.6 (PubChem release 2019.06.18) | |
Source | PubChem | |
URL | https://pubchem.ncbi.nlm.nih.gov | |
Description | Data deposited in or computed by PubChem | |
InChI |
InChI=1S/C12H16O6/c13-6-8-9(14)10(15)11(16)12(18-8)17-7-4-2-1-3-5-7/h1-5,8-16H,6H2/t8-,9+,10+,11-,12+/m1/s1 | |
Details | Computed by InChI 1.0.5 (PubChem release 2019.06.18) | |
Source | PubChem | |
URL | https://pubchem.ncbi.nlm.nih.gov | |
Description | Data deposited in or computed by PubChem | |
InChI Key |
NEZJDVYDSZTRFS-IIRVCBMXSA-N | |
Details | Computed by InChI 1.0.5 (PubChem release 2019.06.18) | |
Source | PubChem | |
URL | https://pubchem.ncbi.nlm.nih.gov | |
Description | Data deposited in or computed by PubChem | |
Canonical SMILES |
C1=CC=C(C=C1)OC2C(C(C(C(O2)CO)O)O)O | |
Details | Computed by OEChem 2.1.5 (PubChem release 2019.06.18) | |
Source | PubChem | |
URL | https://pubchem.ncbi.nlm.nih.gov | |
Description | Data deposited in or computed by PubChem | |
Isomeric SMILES |
C1=CC=C(C=C1)O[C@@H]2[C@@H]([C@H]([C@H]([C@H](O2)CO)O)O)O | |
Details | Computed by OEChem 2.1.5 (PubChem release 2019.06.18) | |
Source | PubChem | |
URL | https://pubchem.ncbi.nlm.nih.gov | |
Description | Data deposited in or computed by PubChem | |
Molecular Formula |
C12H16O6 | |
Details | Computed by PubChem 2.1 (PubChem release 2019.06.18) | |
Source | PubChem | |
URL | https://pubchem.ncbi.nlm.nih.gov | |
Description | Data deposited in or computed by PubChem | |
Molecular Weight |
256.25 g/mol | |
Details | Computed by PubChem 2.1 (PubChem release 2021.05.07) | |
Source | PubChem | |
URL | https://pubchem.ncbi.nlm.nih.gov | |
Description | Data deposited in or computed by PubChem | |
Retrosynthesis Analysis
AI-Powered Synthesis Planning: Our tool employs the Template_relevance Pistachio, Template_relevance Bkms_metabolic, Template_relevance Pistachio_ringbreaker, Template_relevance Reaxys, Template_relevance Reaxys_biocatalysis model, leveraging a vast database of chemical reactions to predict feasible synthetic routes.
One-Step Synthesis Focus: Specifically designed for one-step synthesis, it provides concise and direct routes for your target compounds, streamlining the synthesis process.
Accurate Predictions: Utilizing the extensive PISTACHIO, BKMS_METABOLIC, PISTACHIO_RINGBREAKER, REAXYS, REAXYS_BIOCATALYSIS database, our tool offers high-accuracy predictions, reflecting the latest in chemical research and data.
Strategy Settings
Precursor scoring | Relevance Heuristic |
---|---|
Min. plausibility | 0.01 |
Model | Template_relevance |
Template Set | Pistachio/Bkms_metabolic/Pistachio_ringbreaker/Reaxys/Reaxys_biocatalysis |
Top-N result to add to graph | 6 |
Feasible Synthetic Routes
Disclaimer and Information on In-Vitro Research Products
Please be aware that all articles and product information presented on BenchChem are intended solely for informational purposes. The products available for purchase on BenchChem are specifically designed for in-vitro studies, which are conducted outside of living organisms. In-vitro studies, derived from the Latin term "in glass," involve experiments performed in controlled laboratory settings using cells or tissues. It is important to note that these products are not categorized as medicines or drugs, and they have not received approval from the FDA for the prevention, treatment, or cure of any medical condition, ailment, or disease. We must emphasize that any form of bodily introduction of these products into humans or animals is strictly prohibited by law. It is essential to adhere to these guidelines to ensure compliance with legal and ethical standards in research and experimentation.