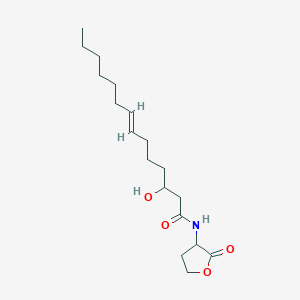
Small bacteriocin
Overview
Description
Small bacteriocins are a subset of ribosomally synthesized antimicrobial peptides, typically characterized by low molecular weight (<10 kDa), heat stability, and narrow-spectrum activity against closely related bacterial species . These peptides are classified under Class II bacteriocins, which lack post-translational modifications such as lanthionine bridges (a hallmark of Class I lantibiotics like nisin) . Examples include rhamnosin A (5.6 kDa) from Lactobacillus rhamnosus and bacteriocin SF1 (≈40 kDa) from Shigella flexneri, both of which exhibit potent activity against Gram-positive pathogens . Their mechanisms often involve membrane disruption via pore formation or enzymatic degradation of cell wall components .
Preparation Methods
Synthetic Routes and Reaction Conditions: Small bacteriocins are typically synthesized through ribosomal pathways in the producing bacteria. The synthesis involves the translation of specific genes encoding the bacteriocin peptides. The peptides are then post-translationally modified to achieve their active forms. Common methods for synthesizing small bacteriocins include:
Fermentation: Bacteria are cultured in nutrient-rich media under controlled conditions to produce bacteriocins.
Industrial Production Methods: Industrial production of small bacteriocins involves large-scale fermentation processes. The bacteria are grown in bioreactors, and the bacteriocins are extracted and purified using advanced chromatographic techniques. The incorporation of bacteriocins into nanoparticles for targeted delivery is also being explored to enhance their effectiveness and minimize potential toxic side effects .
Chemical Reactions Analysis
Key Reactions in Bacteriocin Activity
The activity of small bacteriocins is primarily attributed to their ability to disrupt bacterial cell membranes. The following reactions are critical:
-
Membrane Interaction : Small bacteriocins typically interact with the lipid bilayer of target bacteria, leading to pore formation or membrane disruption. This mechanism is crucial for their antimicrobial activity .
-
Post-Translational Modifications : Many bacteriocins undergo post-translational modifications that enhance their stability and activity. These modifications can include glycosylation or the formation of disulfide bonds, which are essential for maintaining structural integrity .
Stability and Degradation
The stability of small bacteriocins in gastrointestinal conditions has been studied extensively. For example, certain bacteriocins retain their antimicrobial activity under acidic conditions typical of the stomach but may degrade in more neutral environments found in the intestines .
Antimicrobial Activity
Research indicates that small bacteriocins exhibit significant antimicrobial properties against various pathogenic bacteria. Their effectiveness is often measured through agar diffusion assays, where zones of inhibition are observed around wells containing bacteriocin .
Resistance Mechanisms
The emergence of bacterial resistance to bacteriocins has been documented. Studies show that mutations in receptor genes can lead to insensitivity to specific bacteriocins, highlighting the need for combination therapies that utilize multiple bacteriocins to prevent resistance development .
Antimicrobial Activity Against Target Strains
Bacteriocin | Target Strain | Zone of Inhibition (mm) |
---|---|---|
Microcin J25 | Salmonella Newport | 20 |
Nisin Z | Listeria ivanovii | 25 |
Pediocin PA-1 | Staphylococcus aureus | 30 |
Scientific Research Applications
Applications in Food Preservation
Food Safety and Preservation
Bacteriocins are increasingly used as natural preservatives in the food industry. They help extend shelf life by inhibiting spoilage organisms and pathogens. For example:
- Nisin , produced by Lactococcus lactis, is effective against Gram-positive bacteria and is widely used in dairy products and canned foods.
- Pediocin , derived from Pediococcus acidilactici, has shown effectiveness against Listeria monocytogenes in meat products .
Case Study: Nisin in Dairy Products
A study demonstrated that nisin could reduce the growth of Listeria monocytogenes in pasteurized milk, significantly enhancing food safety. The application of nisin at concentrations as low as 10 AU/mL resulted in a 99% reduction of Listeria populations within 24 hours .
Medical Applications
Antimicrobial Agents
Bacteriocins are being explored as alternatives to traditional antibiotics due to their specificity and lower likelihood of resistance development. They have been shown to be effective against multidrug-resistant (MDR) pathogens:
- Enterocin AS-48 has demonstrated activity against Staphylococcus aureus and Enterococcus faecalis, including resistant strains .
- Lacticin 3147 , produced by Lactococcus lactis, exhibits potent activity against various Gram-positive pathogens.
Case Study: Bacteriocins Against Helicobacter pylori
Research indicates that lactic acid bacteria-derived bacteriocins can inhibit Helicobacter pylori, a major cause of peptic ulcers. Bacteriocins such as Lacticin BH5 showed significant inhibitory effects with a minimum inhibitory concentration (MIC) of 12.5 mg/L .
Veterinary Medicine
Bacteriocins are also applied in veterinary medicine to control pathogenic bacteria in livestock. They serve as feed additives that promote gut health and prevent infections.
- Case Study: Use of Pediocin in Poultry
In poultry, the inclusion of pediocin in feed has been shown to reduce the colonization of pathogenic bacteria such as Salmonella and Campylobacter, thereby improving overall animal health and reducing foodborne illness risks .
Agricultural Applications
Biocontrol Agents
In agriculture, small bacteriocins can act as biocontrol agents against plant pathogens. They suppress diseases caused by various bacterial strains, enhancing crop yield and quality.
- Case Study: Rhizobium spp. Bacteriocin
Research on Rhizobium-produced small bacteriocins has demonstrated their efficacy in suppressing bacterial wilt caused by Ralstonia solanacearum. The application of these bacteriocins improved plant resistance and promoted healthier root systems .
Summary Table of Bacteriocin Applications
Mechanism of Action
The mechanism of action of small bacteriocins involves:
Membrane Disruption: Bacteriocins interact with the bacterial cell membrane, causing pore formation and cell lysis.
Cell Wall Biosynthesis Inhibition: Some bacteriocins inhibit the synthesis of bacterial cell walls, leading to cell death.
Targeting Specific Receptors: Bacteriocins bind to specific receptors on the bacterial cell surface, disrupting essential cellular processes
Comparison with Similar Compounds
Comparative Analysis with Similar Bacteriocins
Structural and Functional Classification
Table 1: Structural Comparison of Small Bacteriocin with Other Classes
Small bacteriocins lack the thioether bonds seen in lantibiotics, making their biosynthesis simpler but less stable under extreme conditions . Unlike microcins, which often target intracellular processes, small bacteriocins primarily disrupt membrane integrity, akin to nisin but without requiring lipid II binding .
Production and Purification Efficiency
Table 2: Yield and Purity Across Production Methods
Fusion systems (e.g., lysostaphin catalytic domain) significantly enhance yields compared to native bacterial production, though native systems achieve higher purity . For instance, Lactobacillus pentosus bacteriocin requires optimization to surpass 50% yield in industrial applications .
Antimicrobial Spectrum and Efficacy
Table 3: Activity Against Common Pathogens
Small bacteriocins like rhamnosin A show narrow-spectrum activity, preferentially targeting Gram-positive bacteria, whereas bacteriocin T8 has emerged as critical in hospital-acquired VRE outbreaks . Notably, SF1’s activity against Gram-negative pathogens is rare among Class II bacteriocins and linked to its structural similarity to colicin U, differing by glutamine-to-glutamic acid substitutions .
Stability and Immune Resistance Mechanisms
Key Findings :
- Thermal Stability : Small bacteriocins retain activity after 30 minutes at 65°C but lose efficacy at 80°C, unlike lantibiotics, which withstand autoclaving .
- Immunity Proteins: Producers evade self-inhibition via small transmembrane peptides (e.g., CbnZ in Carnobacterium piscicola), which are genetically distinct from the immunity systems of lantibiotics .
- Plasmid-Mediated Resistance : In S. aureus, immunity is conferred by small plasmids (8–10.4 kb), a feature absent in microcin-producing strains .
Q & A
Basic Research Questions
Q. How to design initial experiments to assess critical factors influencing bacteriocin production?
- Methodology : Use factorial experimental designs to screen variables (e.g., pH, temperature, nutrient concentrations). For example, a 3-level factorial design (e.g., pH 4–8, temperature 20–40°C) can identify significant factors through ANOVA . Measure outputs like bacteriocin titer (AU/mL) and bacterial growth (log CFU/mL) .
- Example : In Lactobacillus sakei studies, glucose concentration (1–10 mg/mL) and Tween 20 (1–20 μL/mL) were systematically varied to isolate their effects on bacteriocin yield .
Q. What methods confirm bacteriocin activity and purity in laboratory settings?
- Methodology :
- Activity assays : Agar diffusion assays against target pathogens (e.g., Listeria monocytogenes) .
- Purity checks : SDS-PAGE for molecular weight confirmation (<14 kDa) and FTIR spectroscopy to detect peptide bonds (e.g., amide I/II peaks at 1,514–1,649 cm⁻¹) .
- Quantification : UV spectrophotometry at 225 nm for peptide concentration .
Q. How to select growth media for maximizing bacteriocin yield in lab cultures?
- Methodology : Compare media like MRS broth, skim milk, or synthetic media with controlled carbon/nitrogen sources. For example, Pediococcus pentosaceus showed optimal bacteriocin production in media with 40 g/L sucrose and 20 g/L peptone . Include pH buffering agents (e.g., phosphate) to stabilize conditions .
Advanced Research Questions
Q. How to optimize bacteriocin production using hybrid modeling (RSM and ANN-GA)?
- Methodology :
- RSM : Central composite design (CCD) to model interactions between variables (e.g., pH, temperature, incubation time). For Lactobacillus plantarum, a CCD predicted optimal conditions at pH 5.05, 20°C, and 18.6 hours .
- ANN-GA : Train artificial neural networks with experimental data to predict yields, then apply genetic algorithms for global optimization. A hybrid model achieved <5% error between predicted and actual bacteriocin yields .
- Validation : Compare adjusted R² values (e.g., RSM R² = 0.9933 vs. ANN R² = 0.997) .
Q. How to resolve contradictory data on bacteriocin activity across studies?
- Methodology :
- Contextual analysis : Compare strain-specific genetic clusters (e.g., immunity genes in BAGEL2 annotations) .
- Experimental replication : Standardize protocols (e.g., agar diffusion assay pH, inoculum size) to minimize variability .
- Meta-analysis : Use databases like Web of Science to aggregate findings and identify trends (e.g., higher activity in low-pH conditions) .
Q. What bioinformatics tools are used for bacteriocin gene mining in genomic data?
- Methodology :
- BAGEL2 : Identifies bacteriocin operons using Hidden Markov Models (HMMs) for conserved domains (e.g., leader peptides) and contextual genes (e.g., transport, immunity) .
- Workflow : Annotate small ORFs (<50 amino acids), screen for antimicrobial motifs, and validate with phylogenomic clustering .
Q. How to address low sample sizes in bacteriocin efficacy studies?
- Methodology :
- Simplified models : Reduce variables (e.g., fix pH at 6.0) to focus on key parameters like temperature .
- Bootstrapping : Resample data to estimate confidence intervals for MIC values .
- Collaborative studies : Pool datasets from multiple labs to increase statistical power .
Q. How to integrate genomic context analysis in bacteriocin mechanism studies?
- Methodology :
- Operon mapping : Use tools like BAGEL2 to link bacteriocin genes to adjacent regulators (e.g., quorum-sensing systems) .
- CRISPR-Cas9 knockouts : Disrupt immunity genes to assess self-protection mechanisms .
Q. Tables for Key Findings
Table 1: Optimized Conditions for Bacteriocin Production
Strain | pH | Temperature (°C) | Key Nutrients | Yield (AU/mL) | Source |
---|---|---|---|---|---|
Lactobacillus sakei | 6.0 | 30 | Glucose (7.75 mg/mL) | 1,024 | |
L. plantarum 1012 | 5.05 | 20 | N/A | 2,400 | |
P. pentosaceus | 7.0 | 30 | Sucrose (40 g/L) | 2,400 |
Table 2: Bioinformatics Tools for Bacteriocin Research
Tool | Function | Example Application | Reference |
---|---|---|---|
BAGEL2 | Genome mining for bacteriocin operons | Identified pediocin in Lactobacillus | |
HMMER | Domain-specific HMM construction | Detected leader peptide motifs |
Properties
IUPAC Name |
(E)-3-hydroxy-N-(2-oxooxolan-3-yl)tetradec-7-enamide | |
---|---|---|
Details | Computed by LexiChem 2.6.6 (PubChem release 2019.06.18) | |
Source | PubChem | |
URL | https://pubchem.ncbi.nlm.nih.gov | |
Description | Data deposited in or computed by PubChem | |
InChI |
InChI=1S/C18H31NO4/c1-2-3-4-5-6-7-8-9-10-11-15(20)14-17(21)19-16-12-13-23-18(16)22/h7-8,15-16,20H,2-6,9-14H2,1H3,(H,19,21)/b8-7+ | |
Details | Computed by InChI 1.0.5 (PubChem release 2019.06.18) | |
Source | PubChem | |
URL | https://pubchem.ncbi.nlm.nih.gov | |
Description | Data deposited in or computed by PubChem | |
InChI Key |
GFSFGXWHLLUVRK-BQYQJAHWSA-N | |
Details | Computed by InChI 1.0.5 (PubChem release 2019.06.18) | |
Source | PubChem | |
URL | https://pubchem.ncbi.nlm.nih.gov | |
Description | Data deposited in or computed by PubChem | |
Canonical SMILES |
CCCCCCC=CCCCC(CC(=O)NC1CCOC1=O)O | |
Details | Computed by OEChem 2.1.5 (PubChem release 2019.06.18) | |
Source | PubChem | |
URL | https://pubchem.ncbi.nlm.nih.gov | |
Description | Data deposited in or computed by PubChem | |
Isomeric SMILES |
CCCCCC/C=C/CCCC(CC(=O)NC1CCOC1=O)O | |
Details | Computed by OEChem 2.1.5 (PubChem release 2019.06.18) | |
Source | PubChem | |
URL | https://pubchem.ncbi.nlm.nih.gov | |
Description | Data deposited in or computed by PubChem | |
Molecular Formula |
C18H31NO4 | |
Details | Computed by PubChem 2.1 (PubChem release 2019.06.18) | |
Source | PubChem | |
URL | https://pubchem.ncbi.nlm.nih.gov | |
Description | Data deposited in or computed by PubChem | |
Molecular Weight |
325.4 g/mol | |
Details | Computed by PubChem 2.1 (PubChem release 2021.05.07) | |
Source | PubChem | |
URL | https://pubchem.ncbi.nlm.nih.gov | |
Description | Data deposited in or computed by PubChem | |
CAS No. |
172617-17-3 | |
Record name | Small bacteriocin | |
Source | Human Metabolome Database (HMDB) | |
URL | http://www.hmdb.ca/metabolites/HMDB0033503 | |
Description | The Human Metabolome Database (HMDB) is a freely available electronic database containing detailed information about small molecule metabolites found in the human body. | |
Explanation | HMDB is offered to the public as a freely available resource. Use and re-distribution of the data, in whole or in part, for commercial purposes requires explicit permission of the authors and explicit acknowledgment of the source material (HMDB) and the original publication (see the HMDB citing page). We ask that users who download significant portions of the database cite the HMDB paper in any resulting publications. | |
Retrosynthesis Analysis
AI-Powered Synthesis Planning: Our tool employs the Template_relevance Pistachio, Template_relevance Bkms_metabolic, Template_relevance Pistachio_ringbreaker, Template_relevance Reaxys, Template_relevance Reaxys_biocatalysis model, leveraging a vast database of chemical reactions to predict feasible synthetic routes.
One-Step Synthesis Focus: Specifically designed for one-step synthesis, it provides concise and direct routes for your target compounds, streamlining the synthesis process.
Accurate Predictions: Utilizing the extensive PISTACHIO, BKMS_METABOLIC, PISTACHIO_RINGBREAKER, REAXYS, REAXYS_BIOCATALYSIS database, our tool offers high-accuracy predictions, reflecting the latest in chemical research and data.
Strategy Settings
Precursor scoring | Relevance Heuristic |
---|---|
Min. plausibility | 0.01 |
Model | Template_relevance |
Template Set | Pistachio/Bkms_metabolic/Pistachio_ringbreaker/Reaxys/Reaxys_biocatalysis |
Top-N result to add to graph | 6 |
Feasible Synthetic Routes
Disclaimer and Information on In-Vitro Research Products
Please be aware that all articles and product information presented on BenchChem are intended solely for informational purposes. The products available for purchase on BenchChem are specifically designed for in-vitro studies, which are conducted outside of living organisms. In-vitro studies, derived from the Latin term "in glass," involve experiments performed in controlled laboratory settings using cells or tissues. It is important to note that these products are not categorized as medicines or drugs, and they have not received approval from the FDA for the prevention, treatment, or cure of any medical condition, ailment, or disease. We must emphasize that any form of bodily introduction of these products into humans or animals is strictly prohibited by law. It is essential to adhere to these guidelines to ensure compliance with legal and ethical standards in research and experimentation.