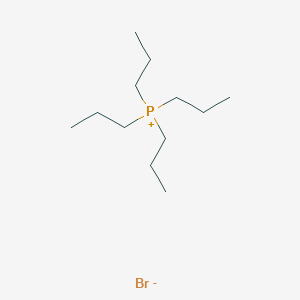
Tetrapropylphosphonium bromide
Overview
Description
Tetrapropylphosphonium bromide is a quaternary phosphonium salt with the chemical formula C12H28BrP. It is a white crystalline solid that is soluble in water and various organic solvents. This compound is widely used in organic synthesis and as a phase-transfer catalyst in various chemical reactions.
Preparation Methods
Synthetic Routes and Reaction Conditions: Tetrapropylphosphonium bromide can be synthesized through the quaternization of tripropylphosphine with propyl bromide. The reaction typically occurs in an organic solvent such as acetonitrile or dichloromethane under reflux conditions. The general reaction is as follows:
P(C3H7)3+C3H7Br→[P(C3H7)4]Br
Industrial Production Methods: In industrial settings, the production of this compound involves the continuous addition of propyl bromide to a solution of tripropylphosphine. The reaction mixture is then heated to facilitate the quaternization process. The product is purified through recrystallization or distillation to achieve high purity levels.
Chemical Reactions Analysis
Types of Reactions: Tetrapropylphosphonium bromide undergoes various chemical reactions, including:
Substitution Reactions: It can participate in nucleophilic substitution reactions where the bromide ion is replaced by other nucleophiles.
Oxidation Reactions: The phosphonium ion can be oxidized to form phosphine oxides.
Coupling Reactions: It is used as a ligand in cross-coupling reactions such as Suzuki-Miyaura, Heck, and Sonogashira couplings.
Common Reagents and Conditions:
Nucleophilic Substitution: Common reagents include sodium hydroxide and potassium carbonate.
Oxidation: Reagents such as hydrogen peroxide or m-chloroperbenzoic acid are used.
Coupling Reactions: Palladium catalysts and bases like potassium carbonate are typically employed.
Major Products:
Substitution: The major products depend on the nucleophile used, such as alkoxides or thiolates.
Oxidation: The primary product is the corresponding phosphine oxide.
Coupling Reactions: The products are often biaryl compounds or other coupled organic molecules.
Scientific Research Applications
Tetrapropylphosphonium bromide has a wide range of applications in scientific research:
Chemistry: It is used as a phase-transfer catalyst to facilitate reactions between aqueous and organic phases. It also serves as a ligand in various metal-catalyzed coupling reactions.
Biology: It is employed in the synthesis of biologically active phosphonium salts that can be used in drug delivery systems.
Medicine: Research is ongoing into its potential use in developing new pharmaceuticals, particularly in targeting mitochondrial functions.
Industry: It is used in the production of specialty chemicals and as an intermediate in the synthesis of other phosphonium salts.
Mechanism of Action
The mechanism by which tetrapropylphosphonium bromide exerts its effects is primarily through its role as a phase-transfer catalyst. It facilitates the transfer of reactants between immiscible phases, thereby increasing the reaction rate. The phosphonium ion interacts with the reactants, stabilizing transition states and lowering activation energy. In biological systems, it targets mitochondrial membranes, affecting their function and integrity.
Comparison with Similar Compounds
Tetrabutylphosphonium Bromide: Similar in structure but with butyl groups instead of propyl groups.
Tetraphenylphosphonium Bromide: Contains phenyl groups, making it more hydrophobic and less soluble in water.
Tetrapropylammonium Bromide: Similar quaternary ammonium salt but with a nitrogen atom instead of phosphorus.
Uniqueness: Tetrapropylphosphonium bromide is unique due to its balance of hydrophobic and hydrophilic properties, making it an effective phase-transfer catalyst. Its ability to participate in a wide range of chemical reactions and its applications in various fields highlight its versatility and importance in scientific research.
Properties
IUPAC Name |
tetrapropylphosphanium;bromide | |
---|---|---|
Details | Computed by LexiChem 2.6.6 (PubChem release 2019.06.18) | |
Source | PubChem | |
URL | https://pubchem.ncbi.nlm.nih.gov | |
Description | Data deposited in or computed by PubChem | |
InChI |
InChI=1S/C12H28P.BrH/c1-5-9-13(10-6-2,11-7-3)12-8-4;/h5-12H2,1-4H3;1H/q+1;/p-1 | |
Details | Computed by InChI 1.0.5 (PubChem release 2019.06.18) | |
Source | PubChem | |
URL | https://pubchem.ncbi.nlm.nih.gov | |
Description | Data deposited in or computed by PubChem | |
InChI Key |
SMGSPBKMKYMJPQ-UHFFFAOYSA-M | |
Details | Computed by InChI 1.0.5 (PubChem release 2019.06.18) | |
Source | PubChem | |
URL | https://pubchem.ncbi.nlm.nih.gov | |
Description | Data deposited in or computed by PubChem | |
Canonical SMILES |
CCC[P+](CCC)(CCC)CCC.[Br-] | |
Details | Computed by OEChem 2.1.5 (PubChem release 2019.06.18) | |
Source | PubChem | |
URL | https://pubchem.ncbi.nlm.nih.gov | |
Description | Data deposited in or computed by PubChem | |
Molecular Formula |
C12H28BrP | |
Details | Computed by PubChem 2.1 (PubChem release 2019.06.18) | |
Source | PubChem | |
URL | https://pubchem.ncbi.nlm.nih.gov | |
Description | Data deposited in or computed by PubChem | |
DSSTOX Substance ID |
DTXSID90451865 | |
Record name | Phosphonium, tetrapropyl-, bromide | |
Source | EPA DSSTox | |
URL | https://comptox.epa.gov/dashboard/DTXSID90451865 | |
Description | DSSTox provides a high quality public chemistry resource for supporting improved predictive toxicology. | |
Molecular Weight |
283.23 g/mol | |
Details | Computed by PubChem 2.1 (PubChem release 2021.05.07) | |
Source | PubChem | |
URL | https://pubchem.ncbi.nlm.nih.gov | |
Description | Data deposited in or computed by PubChem | |
CAS No. |
63462-98-6 | |
Record name | Phosphonium, tetrapropyl-, bromide | |
Source | EPA DSSTox | |
URL | https://comptox.epa.gov/dashboard/DTXSID90451865 | |
Description | DSSTox provides a high quality public chemistry resource for supporting improved predictive toxicology. | |
Synthesis routes and methods
Procedure details
Retrosynthesis Analysis
AI-Powered Synthesis Planning: Our tool employs the Template_relevance Pistachio, Template_relevance Bkms_metabolic, Template_relevance Pistachio_ringbreaker, Template_relevance Reaxys, Template_relevance Reaxys_biocatalysis model, leveraging a vast database of chemical reactions to predict feasible synthetic routes.
One-Step Synthesis Focus: Specifically designed for one-step synthesis, it provides concise and direct routes for your target compounds, streamlining the synthesis process.
Accurate Predictions: Utilizing the extensive PISTACHIO, BKMS_METABOLIC, PISTACHIO_RINGBREAKER, REAXYS, REAXYS_BIOCATALYSIS database, our tool offers high-accuracy predictions, reflecting the latest in chemical research and data.
Strategy Settings
Precursor scoring | Relevance Heuristic |
---|---|
Min. plausibility | 0.01 |
Model | Template_relevance |
Template Set | Pistachio/Bkms_metabolic/Pistachio_ringbreaker/Reaxys/Reaxys_biocatalysis |
Top-N result to add to graph | 6 |
Feasible Synthetic Routes
Disclaimer and Information on In-Vitro Research Products
Please be aware that all articles and product information presented on BenchChem are intended solely for informational purposes. The products available for purchase on BenchChem are specifically designed for in-vitro studies, which are conducted outside of living organisms. In-vitro studies, derived from the Latin term "in glass," involve experiments performed in controlled laboratory settings using cells or tissues. It is important to note that these products are not categorized as medicines or drugs, and they have not received approval from the FDA for the prevention, treatment, or cure of any medical condition, ailment, or disease. We must emphasize that any form of bodily introduction of these products into humans or animals is strictly prohibited by law. It is essential to adhere to these guidelines to ensure compliance with legal and ethical standards in research and experimentation.