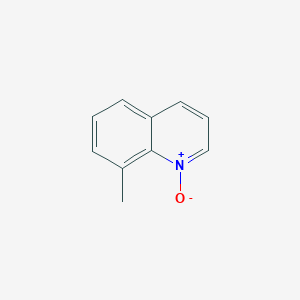
8-Methylquinoline N-oxide
Overview
Description
8-Methylquinoline N-oxide is a heterocyclic aromatic compound with the empirical formula C10H9NO and a molecular weight of 159.18 g/mol . It is a derivative of quinoline, where the nitrogen atom is oxidized to form an N-oxide. This compound is known for its unique chemical properties and is used in various scientific research applications.
Mechanism of Action
Target of Action
8-Methylquinoline N-oxide, also known as 8-methylquinoline 1-oxide, is a compound that primarily targets internal alkynes . Alkynes are unsaturated hydrocarbons containing a triple bond between two carbon atoms. They play a crucial role in various biochemical reactions and pathways.
Mode of Action
The compound interacts with its targets through a process known as gold-catalyzed intermolecular oxidation . This process involves the use of 8-alkylquinoline N-oxides as oxidants and occurs in the absence of acid additives . The interaction results in high regioselectivities , leading to changes in the structure and function of the targeted alkynes.
Biochemical Pathways
The affected biochemical pathway primarily involves the oxidation of internal alkynes . This oxidation process leads to the formation of synthetically versatile α,β-unsaturated carbonyls . These carbonyls are important intermediates in various biochemical reactions and can lead to the production of a wide range of bioactive compounds.
Result of Action
The result of the action of this compound is the production of α,β-unsaturated carbonyls . These carbonyls are synthetically versatile and can be used to produce a wide range of bioactive compounds. The exact molecular and cellular effects would depend on the specific biochemical context and the downstream reactions involving the produced carbonyls.
Action Environment
The action of this compound can be influenced by various environmental factors. For instance, the presence of other reactants, the pH of the environment, and the temperature can all affect the efficiency and stability of the gold-catalyzed intermolecular oxidation process . Understanding these influences can be crucial for optimizing the use of this compound in biochemical applications.
Preparation Methods
Synthetic Routes and Reaction Conditions
8-Methylquinoline N-oxide can be synthesized through several methods. One common approach involves the oxidation of 8-methylquinoline using oxidizing agents such as hydrogen peroxide or peracids . The reaction typically occurs under mild conditions, and the product is purified through recrystallization.
Industrial Production Methods
In industrial settings, the production of this compound often involves large-scale oxidation processes using environmentally friendly oxidants. The use of catalysts and optimized reaction conditions ensures high yield and purity of the final product .
Chemical Reactions Analysis
Types of Reactions
8-Methylquinoline N-oxide undergoes various chemical reactions, including:
Oxidation: It can be further oxidized to form quinoline derivatives.
Reduction: The N-oxide group can be reduced back to the parent quinoline compound.
Substitution: Electrophilic and nucleophilic substitution reactions can occur at different positions on the quinoline ring.
Common Reagents and Conditions
Oxidation: Hydrogen peroxide, peracids.
Reduction: Catalytic hydrogenation, metal hydrides.
Substitution: Halogenating agents, alkylating agents.
Major Products
The major products formed from these reactions include various substituted quinolines and quinoline derivatives, which have significant applications in medicinal chemistry and material science .
Scientific Research Applications
8-Methylquinoline N-oxide is used in a wide range of scientific research applications:
Chemistry: It serves as a building block for the synthesis of more complex heterocyclic compounds.
Biology: It is used in the study of enzyme mechanisms and as a probe for biological systems.
Medicine: Derivatives of this compound have shown potential as anticancer and antimicrobial agents.
Industry: It is used in the production of dyes, pigments, and other industrial chemicals.
Comparison with Similar Compounds
Similar Compounds
- 4-Methylpyridine N-oxide
- 2-Methylpyridine N-oxide
- Isoquinoline N-oxide
- 8-Quinolinol N-oxide
- 6-Methoxyquinoline N-oxide
- 4-Nitroquinoline N-oxide
Uniqueness
8-Methylquinoline N-oxide is unique due to its specific substitution pattern and the presence of the N-oxide group, which imparts distinct chemical reactivity and biological activity. Its derivatives have shown promising applications in various fields, making it a valuable compound for scientific research .
Properties
IUPAC Name |
8-methyl-1-oxidoquinolin-1-ium | |
---|---|---|
Details | Computed by LexiChem 2.6.6 (PubChem release 2019.06.18) | |
Source | PubChem | |
URL | https://pubchem.ncbi.nlm.nih.gov | |
Description | Data deposited in or computed by PubChem | |
InChI |
InChI=1S/C10H9NO/c1-8-4-2-5-9-6-3-7-11(12)10(8)9/h2-7H,1H3 | |
Details | Computed by InChI 1.0.5 (PubChem release 2019.06.18) | |
Source | PubChem | |
URL | https://pubchem.ncbi.nlm.nih.gov | |
Description | Data deposited in or computed by PubChem | |
InChI Key |
PIOGDSHYODXWAK-UHFFFAOYSA-N | |
Details | Computed by InChI 1.0.5 (PubChem release 2019.06.18) | |
Source | PubChem | |
URL | https://pubchem.ncbi.nlm.nih.gov | |
Description | Data deposited in or computed by PubChem | |
Canonical SMILES |
CC1=C2C(=CC=C1)C=CC=[N+]2[O-] | |
Details | Computed by OEChem 2.1.5 (PubChem release 2019.06.18) | |
Source | PubChem | |
URL | https://pubchem.ncbi.nlm.nih.gov | |
Description | Data deposited in or computed by PubChem | |
Molecular Formula |
C10H9NO | |
Details | Computed by PubChem 2.1 (PubChem release 2019.06.18) | |
Source | PubChem | |
URL | https://pubchem.ncbi.nlm.nih.gov | |
Description | Data deposited in or computed by PubChem | |
Molecular Weight |
159.18 g/mol | |
Details | Computed by PubChem 2.1 (PubChem release 2021.05.07) | |
Source | PubChem | |
URL | https://pubchem.ncbi.nlm.nih.gov | |
Description | Data deposited in or computed by PubChem | |
CAS No. |
4053-38-7 | |
Record name | Quinoline, 8-methyl-, 1-oxide | |
Source | CAS Common Chemistry | |
URL | https://commonchemistry.cas.org/detail?cas_rn=4053-38-7 | |
Description | CAS Common Chemistry is an open community resource for accessing chemical information. Nearly 500,000 chemical substances from CAS REGISTRY cover areas of community interest, including common and frequently regulated chemicals, and those relevant to high school and undergraduate chemistry classes. This chemical information, curated by our expert scientists, is provided in alignment with our mission as a division of the American Chemical Society. | |
Explanation | The data from CAS Common Chemistry is provided under a CC-BY-NC 4.0 license, unless otherwise stated. | |
Synthesis routes and methods I
Procedure details
Synthesis routes and methods II
Procedure details
Synthesis routes and methods III
Procedure details
Retrosynthesis Analysis
AI-Powered Synthesis Planning: Our tool employs the Template_relevance Pistachio, Template_relevance Bkms_metabolic, Template_relevance Pistachio_ringbreaker, Template_relevance Reaxys, Template_relevance Reaxys_biocatalysis model, leveraging a vast database of chemical reactions to predict feasible synthetic routes.
One-Step Synthesis Focus: Specifically designed for one-step synthesis, it provides concise and direct routes for your target compounds, streamlining the synthesis process.
Accurate Predictions: Utilizing the extensive PISTACHIO, BKMS_METABOLIC, PISTACHIO_RINGBREAKER, REAXYS, REAXYS_BIOCATALYSIS database, our tool offers high-accuracy predictions, reflecting the latest in chemical research and data.
Strategy Settings
Precursor scoring | Relevance Heuristic |
---|---|
Min. plausibility | 0.01 |
Model | Template_relevance |
Template Set | Pistachio/Bkms_metabolic/Pistachio_ringbreaker/Reaxys/Reaxys_biocatalysis |
Top-N result to add to graph | 6 |
Feasible Synthetic Routes
Disclaimer and Information on In-Vitro Research Products
Please be aware that all articles and product information presented on BenchChem are intended solely for informational purposes. The products available for purchase on BenchChem are specifically designed for in-vitro studies, which are conducted outside of living organisms. In-vitro studies, derived from the Latin term "in glass," involve experiments performed in controlled laboratory settings using cells or tissues. It is important to note that these products are not categorized as medicines or drugs, and they have not received approval from the FDA for the prevention, treatment, or cure of any medical condition, ailment, or disease. We must emphasize that any form of bodily introduction of these products into humans or animals is strictly prohibited by law. It is essential to adhere to these guidelines to ensure compliance with legal and ethical standards in research and experimentation.