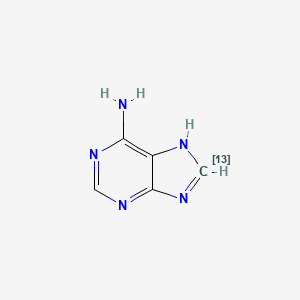
Adenine-13C
Overview
Description
Adenine-13C is a stable isotope-labeled compound where the carbon-13 isotope is incorporated into the adenine moleculeIt plays a crucial role in cellular respiration, forming adenosine triphosphate (ATP), and is involved in the synthesis of nucleotides and nucleic acids .
Preparation Methods
Synthetic Routes and Reaction Conditions
The synthesis of Adenine-13C typically involves the incorporation of the carbon-13 isotope into the adenine molecule. One common method is the chemo-enzymatic synthesis, which uses labeled precursors to introduce the isotope into the desired position within the molecule . This method ensures high specificity and yield.
Industrial Production Methods
Industrial production of this compound involves large-scale synthesis using labeled carbon sources. The process includes the fermentation of microorganisms in a medium enriched with carbon-13 labeled substrates. The labeled adenine is then extracted and purified using various chromatographic techniques .
Chemical Reactions Analysis
Types of Reactions
Adenine-13C undergoes several types of chemical reactions, including:
Oxidation: Adenine can be oxidized to form 8-oxoadenine, a common oxidative lesion in DNA.
Reduction: Reduction reactions can convert adenine to dihydroadenine.
Substitution: Adenine can participate in substitution reactions, where functional groups are replaced by other groups.
Common Reagents and Conditions
Oxidation: Hydrogen peroxide or potassium permanganate under acidic conditions.
Reduction: Sodium borohydride or lithium aluminum hydride in anhydrous solvents.
Substitution: Halogenating agents like chlorine or bromine in the presence of a catalyst.
Major Products Formed
Oxidation: 8-oxoadenine.
Reduction: Dihydroadenine.
Substitution: Halogenated adenine derivatives.
Scientific Research Applications
Adenine-13C has numerous applications in scientific research:
Chemistry: Used as a tracer in nuclear magnetic resonance (NMR) spectroscopy to study molecular structures and dynamics.
Biology: Helps in understanding metabolic pathways and nucleotide synthesis.
Medicine: Used in drug development and pharmacokinetics to track the metabolism of adenine-containing drugs.
Industry: Employed in the production of labeled nucleotides for research and diagnostic purposes.
Mechanism of Action
Adenine-13C exerts its effects by participating in various biochemical pathways. It forms adenosine when attached to ribose and deoxyadenosine when attached to deoxyribose. These nucleosides are essential for the synthesis of ATP, which drives many cellular metabolic processes by transferring chemical energy between reactions. This compound also plays a role in the formation of cofactors like nicotinamide adenine dinucleotide (NAD) and flavin adenine dinucleotide (FAD), which are crucial for cellular respiration .
Comparison with Similar Compounds
Similar Compounds
Guanine-13C: Another labeled nucleobase used in similar applications.
Cytosine-13C: Used in studies of DNA and RNA synthesis.
Thymine-13C: Employed in research on DNA replication and repair.
Uniqueness
Adenine-13C is unique due to its specific role in ATP synthesis and its involvement in both DNA and RNA. Its stable isotope labeling allows for precise tracking and analysis in various biochemical and pharmacological studies, making it a valuable tool in research .
Properties
IUPAC Name |
7H-purin-6-amine | |
---|---|---|
Details | Computed by Lexichem TK 2.7.0 (PubChem release 2021.05.07) | |
Source | PubChem | |
URL | https://pubchem.ncbi.nlm.nih.gov | |
Description | Data deposited in or computed by PubChem | |
InChI |
InChI=1S/C5H5N5/c6-4-3-5(9-1-7-3)10-2-8-4/h1-2H,(H3,6,7,8,9,10)/i1+1 | |
Details | Computed by InChI 1.0.6 (PubChem release 2021.05.07) | |
Source | PubChem | |
URL | https://pubchem.ncbi.nlm.nih.gov | |
Description | Data deposited in or computed by PubChem | |
InChI Key |
GFFGJBXGBJISGV-OUBTZVSYSA-N | |
Details | Computed by InChI 1.0.6 (PubChem release 2021.05.07) | |
Source | PubChem | |
URL | https://pubchem.ncbi.nlm.nih.gov | |
Description | Data deposited in or computed by PubChem | |
Canonical SMILES |
C1=NC2=NC=NC(=C2N1)N | |
Details | Computed by OEChem 2.3.0 (PubChem release 2021.05.07) | |
Source | PubChem | |
URL | https://pubchem.ncbi.nlm.nih.gov | |
Description | Data deposited in or computed by PubChem | |
Isomeric SMILES |
C1=NC(=C2C(=N1)N=[13CH]N2)N | |
Details | Computed by OEChem 2.3.0 (PubChem release 2021.05.07) | |
Source | PubChem | |
URL | https://pubchem.ncbi.nlm.nih.gov | |
Description | Data deposited in or computed by PubChem | |
Molecular Formula |
C5H5N5 | |
Details | Computed by PubChem 2.1 (PubChem release 2021.05.07) | |
Source | PubChem | |
URL | https://pubchem.ncbi.nlm.nih.gov | |
Description | Data deposited in or computed by PubChem | |
Molecular Weight |
136.12 g/mol | |
Details | Computed by PubChem 2.1 (PubChem release 2021.05.07) | |
Source | PubChem | |
URL | https://pubchem.ncbi.nlm.nih.gov | |
Description | Data deposited in or computed by PubChem | |
Synthesis routes and methods I
Procedure details
Synthesis routes and methods II
Procedure details
Synthesis routes and methods III
Procedure details
Retrosynthesis Analysis
AI-Powered Synthesis Planning: Our tool employs the Template_relevance Pistachio, Template_relevance Bkms_metabolic, Template_relevance Pistachio_ringbreaker, Template_relevance Reaxys, Template_relevance Reaxys_biocatalysis model, leveraging a vast database of chemical reactions to predict feasible synthetic routes.
One-Step Synthesis Focus: Specifically designed for one-step synthesis, it provides concise and direct routes for your target compounds, streamlining the synthesis process.
Accurate Predictions: Utilizing the extensive PISTACHIO, BKMS_METABOLIC, PISTACHIO_RINGBREAKER, REAXYS, REAXYS_BIOCATALYSIS database, our tool offers high-accuracy predictions, reflecting the latest in chemical research and data.
Strategy Settings
Precursor scoring | Relevance Heuristic |
---|---|
Min. plausibility | 0.01 |
Model | Template_relevance |
Template Set | Pistachio/Bkms_metabolic/Pistachio_ringbreaker/Reaxys/Reaxys_biocatalysis |
Top-N result to add to graph | 6 |
Feasible Synthetic Routes
Disclaimer and Information on In-Vitro Research Products
Please be aware that all articles and product information presented on BenchChem are intended solely for informational purposes. The products available for purchase on BenchChem are specifically designed for in-vitro studies, which are conducted outside of living organisms. In-vitro studies, derived from the Latin term "in glass," involve experiments performed in controlled laboratory settings using cells or tissues. It is important to note that these products are not categorized as medicines or drugs, and they have not received approval from the FDA for the prevention, treatment, or cure of any medical condition, ailment, or disease. We must emphasize that any form of bodily introduction of these products into humans or animals is strictly prohibited by law. It is essential to adhere to these guidelines to ensure compliance with legal and ethical standards in research and experimentation.