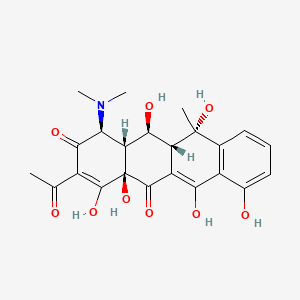
Terramycin-X
Overview
Description
Mechanism of Action
Target of Action
Terramycin-X primarily targets the 30S ribosomal subunit of bacteria . This subunit is a crucial component of the bacterial protein synthesis machinery. By binding to this subunit, this compound can effectively inhibit protein synthesis, thereby preventing bacterial growth .
Mode of Action
This compound inhibits bacterial growth by preventing the amino-acyl tRNA from binding to the A site of the ribosome . This binding is reversible in nature . By blocking this critical step in protein synthesis, this compound prevents the bacteria from producing essential proteins, thereby inhibiting their growth .
Biochemical Pathways
The primary biochemical pathway affected by this compound is the protein synthesis pathway in bacteria . By binding to the 30S ribosomal subunit, this compound prevents the proper functioning of this pathway, leading to a halt in protein production and thus bacterial growth .
Result of Action
The primary result of this compound’s action is the inhibition of bacterial growth . By preventing protein synthesis, this compound effectively halts the growth of bacteria, making it a potent antibiotic for treating a variety of bacterial infections .
Action Environment
Environmental factors can significantly influence the action, efficacy, and stability of this compound. For instance, after medication, more than 70% of tetracycline antibiotics like this compound are excreted and released in active form into the environment via urine and feces from humans and animals . Their highly hydrophilic character and low volatility result in significant persistence in the aquatic environment . The presence of such drugs in the environment could affect the growth of some terrestrial and aquatic species .
Biochemical Analysis
Biochemical Properties
Terramycin-X plays a significant role in biochemical reactions. It inhibits bacterial growth by preventing protein biosynthesis . It achieves this by binding to the 30S ribosomal subunit, which prevents the amino-acyl tRNA from binding to the A site of the ribosome . This interaction disrupts the protein synthesis process, thereby inhibiting bacterial growth .
Cellular Effects
This compound has a wide range of effects on various types of cells and cellular processes. It is effective against both gram-positive and gram-negative bacteria, as well as intracellular chlamydiae, mycoplasmas, and rickettsiae . It influences cell function by inhibiting protein biosynthesis, which is a crucial process for cell growth and replication .
Molecular Mechanism
The molecular mechanism of this compound involves its binding to the 30S ribosomal subunit, which prevents the amino-acyl tRNA from binding to the A site of the ribosome . This interaction disrupts the protein synthesis process, thereby inhibiting bacterial growth .
Temporal Effects in Laboratory Settings
In laboratory settings, the effects of this compound can change over time. For instance, heat inactivation of this compound in reconstituted milk of different solids concentrations was found to be inversely related to the solids content . During storage, this compound lost its potency at a faster rate in the unheated system than in the heated system .
Dosage Effects in Animal Models
In animal models, the effects of this compound can vary with different dosages. For instance, calves were treated with this compound at a dose of 20 mg/kg, and no significant differences were found between the two treatment groups with regard to the number of injections needed for a complete cure .
Metabolic Pathways
This compound is involved in several metabolic pathways. It is derived from acetyl-CoA and malonyl CoA, which are key intermediates in many metabolic pathways . The overproducer exhibited increased acetyl-CoA and malonyl CoA supply, upregulated oxytetracycline biosynthesis, reduced competing byproduct formation, and streamlined morphology .
Transport and Distribution
The transport and distribution of this compound within cells and tissues are complex processes. Antibiotics transport in the environment is associated with its physico-chemical properties. The transport of antibiotics in soils is mainly controlled by the sorptivity, life time, soil solution pH, and ionic strength .
Subcellular Localization
It is known that the subcellular location of a protein provides valuable information about its functionalities, the functioning of the cell, and its possible interactions with other proteins .
Preparation Methods
Synthetic Routes and Reaction Conditions: Terramycin-X is produced through the fermentation of Streptomyces rimosus. The process involves culturing the bacterium in a nutrient-rich medium, followed by extraction and purification of the antibiotic. The initial isolation involves solvent extraction and precipitation of mixed inorganic salts . Chromatography techniques, such as paper partition chromatography, are used to further purify the compound .
Industrial Production Methods: Industrial production of this compound relies on the fermentation process using hyperproducing strains of Streptomyces rimosus. These strains are often mutagenized to enhance production yields. The fermentation broth is subjected to solvent extraction, followed by purification steps involving chromatography and crystallization to obtain pure oxytetracycline .
Chemical Reactions Analysis
Types of Reactions: Terramycin-X undergoes various chemical reactions, including oxidation, reduction, and substitution. For instance, it can be oxidized to form different derivatives, and it can also undergo substitution reactions to form new compounds .
Common Reagents and Conditions: Common reagents used in the reactions of this compound include hydrochloric acid, sodium hydride, and various organic solvents. The conditions for these reactions typically involve controlled temperatures and pH levels to ensure the desired transformations .
Major Products: The major products formed from the reactions of this compound include its derivatives, such as chlortetracycline and doxycycline. These derivatives are often synthesized to enhance the antibiotic properties or to reduce resistance .
Scientific Research Applications
Terramycin-X has a wide range of applications in scientific research:
Comparison with Similar Compounds
Chlortetracycline: Another tetracycline antibiotic with similar broad-spectrum activity.
Doxycycline: A derivative of tetracycline with improved pharmacokinetic properties.
Minocycline: Known for its enhanced ability to penetrate tissues and its effectiveness against resistant strains
Uniqueness: Terramycin-X is unique due to its broad-spectrum activity and its ability to penetrate deep into tissues, making it effective against a wide range of infections. Its discovery paved the way for the development of other tetracycline antibiotics, and it remains a valuable tool in both human and veterinary medicine .
Properties
IUPAC Name |
(1S,4aR,11S,11aR,12S,12aR)-3-acetyl-1-(dimethylamino)-4,4a,6,7,11,12-hexahydroxy-11-methyl-1,11a,12,12a-tetrahydrotetracene-2,5-dione | |
---|---|---|
Details | Computed by Lexichem TK 2.7.0 (PubChem release 2021.05.07) | |
Source | PubChem | |
URL | https://pubchem.ncbi.nlm.nih.gov | |
Description | Data deposited in or computed by PubChem | |
InChI |
InChI=1S/C23H25NO9/c1-8(25)11-18(28)16(24(3)4)15-19(29)14-13(21(31)23(15,33)20(11)30)17(27)12-9(22(14,2)32)6-5-7-10(12)26/h5-7,14-16,19,26-27,29-30,32-33H,1-4H3/t14-,15-,16+,19+,22-,23-/m1/s1 | |
Details | Computed by InChI 1.0.6 (PubChem release 2021.05.07) | |
Source | PubChem | |
URL | https://pubchem.ncbi.nlm.nih.gov | |
Description | Data deposited in or computed by PubChem | |
InChI Key |
IEXLMWZQCURUMB-JSILLTCQSA-N | |
Details | Computed by InChI 1.0.6 (PubChem release 2021.05.07) | |
Source | PubChem | |
URL | https://pubchem.ncbi.nlm.nih.gov | |
Description | Data deposited in or computed by PubChem | |
Canonical SMILES |
CC(=O)C1=C(C2(C(C(C3C(=C(C4=C(C3(C)O)C=CC=C4O)O)C2=O)O)C(C1=O)N(C)C)O)O | |
Details | Computed by OEChem 2.3.0 (PubChem release 2021.05.07) | |
Source | PubChem | |
URL | https://pubchem.ncbi.nlm.nih.gov | |
Description | Data deposited in or computed by PubChem | |
Isomeric SMILES |
CC(=O)C1=C([C@@]2([C@@H]([C@H]([C@H]3C(=C(C4=C([C@@]3(C)O)C=CC=C4O)O)C2=O)O)[C@@H](C1=O)N(C)C)O)O | |
Details | Computed by OEChem 2.3.0 (PubChem release 2021.05.07) | |
Source | PubChem | |
URL | https://pubchem.ncbi.nlm.nih.gov | |
Description | Data deposited in or computed by PubChem | |
Molecular Formula |
C23H25NO9 | |
Details | Computed by PubChem 2.1 (PubChem release 2021.05.07) | |
Source | PubChem | |
URL | https://pubchem.ncbi.nlm.nih.gov | |
Description | Data deposited in or computed by PubChem | |
DSSTOX Substance ID |
DTXSID801101239 | |
Record name | (4S,4aR,5S,5aR,6S,12aS)-2-Acetyl-4-(dimethylamino)-4a,5a,6,12a-tetrahydro-3,5,6,10,12,12a-hexahydroxy-6-methyl-1,11(4H,5H)-naphthacenedione | |
Source | EPA DSSTox | |
URL | https://comptox.epa.gov/dashboard/DTXSID801101239 | |
Description | DSSTox provides a high quality public chemistry resource for supporting improved predictive toxicology. | |
Molecular Weight |
459.4 g/mol | |
Details | Computed by PubChem 2.1 (PubChem release 2021.05.07) | |
Source | PubChem | |
URL | https://pubchem.ncbi.nlm.nih.gov | |
Description | Data deposited in or computed by PubChem | |
CAS No. |
7647-65-6 | |
Record name | Oxytetracycline, 2-acetyl-2-de(aminocarbonyl)- | |
Source | ChemIDplus | |
URL | https://pubchem.ncbi.nlm.nih.gov/substance/?source=chemidplus&sourceid=0007647656 | |
Description | ChemIDplus is a free, web search system that provides access to the structure and nomenclature authority files used for the identification of chemical substances cited in National Library of Medicine (NLM) databases, including the TOXNET system. | |
Record name | (4S,4aR,5S,5aR,6S,12aS)-2-Acetyl-4-(dimethylamino)-4a,5a,6,12a-tetrahydro-3,5,6,10,12,12a-hexahydroxy-6-methyl-1,11(4H,5H)-naphthacenedione | |
Source | EPA DSSTox | |
URL | https://comptox.epa.gov/dashboard/DTXSID801101239 | |
Description | DSSTox provides a high quality public chemistry resource for supporting improved predictive toxicology. | |
Record name | 2-ACETYL-2-DECARBAMOYLOXYTETRACYCLINE | |
Source | FDA Global Substance Registration System (GSRS) | |
URL | https://gsrs.ncats.nih.gov/ginas/app/beta/substances/NNV80I68DM | |
Description | The FDA Global Substance Registration System (GSRS) enables the efficient and accurate exchange of information on what substances are in regulated products. Instead of relying on names, which vary across regulatory domains, countries, and regions, the GSRS knowledge base makes it possible for substances to be defined by standardized, scientific descriptions. | |
Explanation | Unless otherwise noted, the contents of the FDA website (www.fda.gov), both text and graphics, are not copyrighted. They are in the public domain and may be republished, reprinted and otherwise used freely by anyone without the need to obtain permission from FDA. Credit to the U.S. Food and Drug Administration as the source is appreciated but not required. | |
Retrosynthesis Analysis
AI-Powered Synthesis Planning: Our tool employs the Template_relevance Pistachio, Template_relevance Bkms_metabolic, Template_relevance Pistachio_ringbreaker, Template_relevance Reaxys, Template_relevance Reaxys_biocatalysis model, leveraging a vast database of chemical reactions to predict feasible synthetic routes.
One-Step Synthesis Focus: Specifically designed for one-step synthesis, it provides concise and direct routes for your target compounds, streamlining the synthesis process.
Accurate Predictions: Utilizing the extensive PISTACHIO, BKMS_METABOLIC, PISTACHIO_RINGBREAKER, REAXYS, REAXYS_BIOCATALYSIS database, our tool offers high-accuracy predictions, reflecting the latest in chemical research and data.
Strategy Settings
Precursor scoring | Relevance Heuristic |
---|---|
Min. plausibility | 0.01 |
Model | Template_relevance |
Template Set | Pistachio/Bkms_metabolic/Pistachio_ringbreaker/Reaxys/Reaxys_biocatalysis |
Top-N result to add to graph | 6 |
Feasible Synthetic Routes
Disclaimer and Information on In-Vitro Research Products
Please be aware that all articles and product information presented on BenchChem are intended solely for informational purposes. The products available for purchase on BenchChem are specifically designed for in-vitro studies, which are conducted outside of living organisms. In-vitro studies, derived from the Latin term "in glass," involve experiments performed in controlled laboratory settings using cells or tissues. It is important to note that these products are not categorized as medicines or drugs, and they have not received approval from the FDA for the prevention, treatment, or cure of any medical condition, ailment, or disease. We must emphasize that any form of bodily introduction of these products into humans or animals is strictly prohibited by law. It is essential to adhere to these guidelines to ensure compliance with legal and ethical standards in research and experimentation.