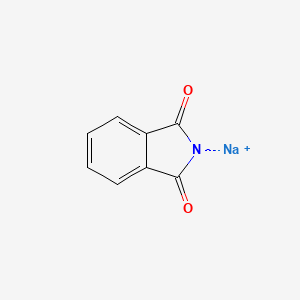
Sodium phthalimide
Overview
Description
Sodium phthalimide is an organic compound derived from phthalimide, where the hydrogen atom of the imide group is replaced by a sodium ion. It is commonly used as a reagent in organic synthesis, particularly in the Gabriel synthesis of primary amines. This compound is a white crystalline solid that is soluble in water and organic solvents.
Mechanism of Action
Target of Action
Sodium phthalimide is a derivative of phthalimide, which is a privileged structural motif frequently found in natural products, pharmaceuticals, and organic materials .
Mode of Action
This compound is readily deprotonated by bases such as sodium hydroxide to give the corresponding anion . This anion can then react with electrophiles such as alkyl halides in a process known as the Gabriel Synthesis . The high acidity of the imido N-H is the result of the pair of flanking electrophilic carbonyl groups .
Biochemical Pathways
For instance, they can undergo carbonylative cyclization of aromatic amides, carbonylative cyclization of o-dihaloarenes/o-haloarenes, cyclization of isocyanate/isocyanide with arenes, and cyclization involving maleimides .
Result of Action
For instance, some phthalimides have been found to exhibit antiproliferative activity against cancer cells .
Action Environment
The action of this compound can be influenced by various environmental factors. For instance, the pH of the environment can affect the deprotonation of this compound . Additionally, the presence of other chemical species in the environment can influence the reactivity of this compound .
Biochemical Analysis
Biochemical Properties
Sodium phthalimide plays a role in biochemical reactions. It is used as a precursor to other organic compounds, indicating that it interacts with various enzymes, proteins, and other biomolecules
Cellular Effects
Related compounds, such as phthalimide derivatives, have been shown to exhibit antineoplastic activities against cancer cells This suggests that this compound may influence cell function, potentially impacting cell signaling pathways, gene expression, and cellular metabolism
Molecular Mechanism
It is known to form salts upon treatment with bases such as sodium hydroxide . The high acidity of the imido N-H is the result of the pair of flanking electrophilic carbonyl groups This suggests that this compound may interact with biomolecules through binding interactions, potentially influencing enzyme activity and gene expression
Metabolic Pathways
This compound is involved in metabolic pathways as a precursor to other organic compounds
Preparation Methods
Synthetic Routes and Reaction Conditions: Sodium phthalimide can be synthesized by reacting phthalimide with sodium hydroxide. The reaction involves the deprotonation of phthalimide by sodium hydroxide, resulting in the formation of this compound and water:
C8H5NO2+NaOH→C8H4NO2Na+H2O
Industrial Production Methods: In industrial settings, this compound is produced by heating phthalimide with an excess of sodium hydroxide in an aqueous solution. The reaction is typically carried out at elevated temperatures to ensure complete conversion of phthalimide to this compound.
Types of Reactions:
Nucleophilic Substitution: this compound is a strong nucleophile and can undergo nucleophilic substitution reactions with alkyl halides to form N-alkylphthalimides. This reaction is the basis of the Gabriel synthesis for producing primary amines.
Reduction: this compound can be reduced to phthalimide using reducing agents such as lithium aluminum hydride.
Hydrolysis: this compound can be hydrolyzed to phthalic acid and ammonia under acidic conditions.
Common Reagents and Conditions:
Nucleophilic Substitution: Alkyl halides (e.g., methyl iodide) and this compound in an aprotic solvent such as dimethylformamide.
Reduction: Lithium aluminum hydride in anhydrous ether.
Hydrolysis: Dilute hydrochloric acid or sulfuric acid.
Major Products:
N-alkylphthalimides: Formed from nucleophilic substitution reactions.
Phthalimide: Formed from reduction reactions.
Phthalic acid and ammonia: Formed from hydrolysis reactions.
Scientific Research Applications
Sodium phthalimide has a wide range of applications in scientific research:
Chemistry: It is used as a reagent in organic synthesis, particularly in the Gabriel synthesis of primary amines. It is also used in the preparation of various N-substituted phthalimides.
Biology: this compound derivatives have been studied for their potential biological activities, including antimicrobial and anticancer properties.
Medicine: Some this compound derivatives are used in the development of pharmaceuticals, particularly as intermediates in the synthesis of drugs.
Industry: this compound is used in the production of dyes, pigments, and other industrial chemicals.
Comparison with Similar Compounds
- Potassium phthalimide
- Lithium phthalimide
- N-alkylphthalimides
Properties
IUPAC Name |
sodium;isoindol-2-ide-1,3-dione | |
---|---|---|
Details | Computed by Lexichem TK 2.7.0 (PubChem release 2021.05.07) | |
Source | PubChem | |
URL | https://pubchem.ncbi.nlm.nih.gov | |
Description | Data deposited in or computed by PubChem | |
InChI |
InChI=1S/C8H5NO2.Na/c10-7-5-3-1-2-4-6(5)8(11)9-7;/h1-4H,(H,9,10,11);/q;+1/p-1 | |
Details | Computed by InChI 1.0.6 (PubChem release 2021.05.07) | |
Source | PubChem | |
URL | https://pubchem.ncbi.nlm.nih.gov | |
Description | Data deposited in or computed by PubChem | |
InChI Key |
WUPVYJJCKYSGCR-UHFFFAOYSA-M | |
Details | Computed by InChI 1.0.6 (PubChem release 2021.05.07) | |
Source | PubChem | |
URL | https://pubchem.ncbi.nlm.nih.gov | |
Description | Data deposited in or computed by PubChem | |
Canonical SMILES |
C1=CC=C2C(=C1)C(=O)[N-]C2=O.[Na+] | |
Details | Computed by OEChem 2.3.0 (PubChem release 2021.05.07) | |
Source | PubChem | |
URL | https://pubchem.ncbi.nlm.nih.gov | |
Description | Data deposited in or computed by PubChem | |
Molecular Formula |
C8H4NNaO2 | |
Details | Computed by PubChem 2.1 (PubChem release 2021.05.07) | |
Source | PubChem | |
URL | https://pubchem.ncbi.nlm.nih.gov | |
Description | Data deposited in or computed by PubChem | |
Molecular Weight |
169.11 g/mol | |
Details | Computed by PubChem 2.1 (PubChem release 2021.05.07) | |
Source | PubChem | |
URL | https://pubchem.ncbi.nlm.nih.gov | |
Description | Data deposited in or computed by PubChem | |
Retrosynthesis Analysis
AI-Powered Synthesis Planning: Our tool employs the Template_relevance Pistachio, Template_relevance Bkms_metabolic, Template_relevance Pistachio_ringbreaker, Template_relevance Reaxys, Template_relevance Reaxys_biocatalysis model, leveraging a vast database of chemical reactions to predict feasible synthetic routes.
One-Step Synthesis Focus: Specifically designed for one-step synthesis, it provides concise and direct routes for your target compounds, streamlining the synthesis process.
Accurate Predictions: Utilizing the extensive PISTACHIO, BKMS_METABOLIC, PISTACHIO_RINGBREAKER, REAXYS, REAXYS_BIOCATALYSIS database, our tool offers high-accuracy predictions, reflecting the latest in chemical research and data.
Strategy Settings
Precursor scoring | Relevance Heuristic |
---|---|
Min. plausibility | 0.01 |
Model | Template_relevance |
Template Set | Pistachio/Bkms_metabolic/Pistachio_ringbreaker/Reaxys/Reaxys_biocatalysis |
Top-N result to add to graph | 6 |
Feasible Synthetic Routes
Q1: What is the primary application of sodium phthalimide in organic synthesis, as highlighted in the research?
A1: The research emphasizes the use of this compound as a reagent for introducing nitrogen into organic molecules. Specifically, it acts as a synthetic equivalent for ammonia in Gabriel synthesis, facilitating the preparation of primary amines. [, ] This is exemplified by its reaction with 4-chloromethyl styrene copolymers, leading to the incorporation of amino groups into the polymer structure. [] Additionally, this compound demonstrates reactivity with various electrophiles like alkyl halides and aziridinium intermediates, further highlighting its versatility in amine synthesis. [, ]
Q2: Can you elaborate on the catalytic properties of this compound, particularly in the context of dihydropyrano[2,3-g] chromene synthesis?
A2: While this compound is commonly known as a reagent in Gabriel synthesis, one of the papers explores its catalytic potential. [] It acts as a catalyst in conjunction with 1-butyl-3-methylimidazolium tetrafluoroborate ([BMIM]BF4) ionic liquid in the one-pot synthesis of dihydropyrano[2,3-g] chromenes. This method utilizes readily available starting materials like aldehydes, malononitrile, and 2,5-dihydroxy-1,4-benzoquinone. The described benefits include mild reaction conditions, ease of product isolation, high yields, and the potential for large-scale synthesis. []
Q3: How does the structure of phthalimide derivatives relate to their reactivity with vinylic halides?
A3: Research indicates that copper-catalyzed Gabriel reactions, typically employed for aryl halides, can be extended to vinylic halides using this compound. [] The study also explores the reactivity of sodium salts derived from other imide analogs like succinimide, maleimide, and dibenzamide. While these analogs successfully form N-phenyl derivatives upon reaction with vinylic halides, they exhibit lower reactivity compared to this compound. This observation suggests that the specific structure of the phthalimide ring contributes to the enhanced reactivity observed with this compound. [] Further research could elucidate the precise structural features responsible for this difference in reactivity.
Q4: Are there any insights into the analytical techniques used to characterize this compound and its reaction products?
A4: The research papers highlight a range of analytical methods used to characterize this compound derivatives and the products formed in its reactions. Nuclear Magnetic Resonance (NMR) spectroscopy, specifically 1H NMR and 13C NMR, is extensively employed for structural elucidation. [, ] Additionally, Fourier Transform Infrared (FTIR) spectroscopy provides information about functional groups present in the synthesized compounds. [, ] Mass spectrometry and elemental analysis are also mentioned as techniques for confirming molecular weight and elemental composition. [] For polymeric materials, Gel Permeation Chromatography (GPC) is used to determine the molecular weight distribution, while Dynamic Mechanical Thermal Analysis (DMTA) provides insights into the glass transition temperature, which is indicative of the material's physical properties. []
Disclaimer and Information on In-Vitro Research Products
Please be aware that all articles and product information presented on BenchChem are intended solely for informational purposes. The products available for purchase on BenchChem are specifically designed for in-vitro studies, which are conducted outside of living organisms. In-vitro studies, derived from the Latin term "in glass," involve experiments performed in controlled laboratory settings using cells or tissues. It is important to note that these products are not categorized as medicines or drugs, and they have not received approval from the FDA for the prevention, treatment, or cure of any medical condition, ailment, or disease. We must emphasize that any form of bodily introduction of these products into humans or animals is strictly prohibited by law. It is essential to adhere to these guidelines to ensure compliance with legal and ethical standards in research and experimentation.