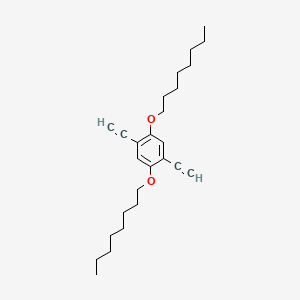
1,4-Diethynyl-2,5-bis(octyloxy)benzene
Overview
Description
1,4-Diethynyl-2,5-bis(octyloxy)benzene (C₂₆H₃₈O₂, molecular mass 382.588 g/mol) is a π-conjugated aromatic compound featuring two ethynyl (-C≡C-) groups at the 1,4-positions and two octyloxy (-O-C₈H₁₇) chains at the 2,5-positions of the benzene ring . The octyloxy substituents enhance solubility in organic solvents while maintaining structural rigidity, making the compound a versatile building block for conjugated polymers and molecular electronics . Its synthesis typically involves a Sonogashira-Hagihara coupling reaction between 2,5-diiodo-1,4-bis(octyloxy)benzene and terminal alkynes, catalyzed by Pd(PPh₃)₂Cl₂ and CuI . Characterization via ¹H NMR, ¹³C NMR, and FT-IR confirms the structural integrity of the product .
Preparation Methods
Synthetic Routes and Reaction Conditions
1,4-Diethynyl-2,5-bis(octyloxy)benzene can be synthesized through a multi-step process involving the following key steps:
Formation of the Benzene Core: The benzene core is initially substituted with octyloxy groups at the 2 and 5 positions.
Introduction of Ethynyl Groups: The ethynyl groups are introduced via a Sonogashira coupling reaction, which involves the reaction of a halogenated benzene derivative with an acetylene compound in the presence of a palladium catalyst and a copper co-catalyst.
Industrial Production Methods
While specific industrial production methods for this compound are not widely documented, the general approach involves scaling up the laboratory synthesis process. This includes optimizing reaction conditions such as temperature, pressure, and catalyst concentration to achieve higher yields and purity .
Chemical Reactions Analysis
Types of Reactions
1,4-Diethynyl-2,5-bis(octyloxy)benzene undergoes various chemical reactions, including:
Coupling Reactions: It participates in Sonogashira coupling reactions to form conjugated polymers.
Oxidation and Reduction: The ethynyl groups can undergo oxidation and reduction reactions under appropriate conditions.
Substitution Reactions: The octyloxy groups can be substituted with other functional groups through nucleophilic substitution reactions.
Common Reagents and Conditions
Sonogashira Coupling: Palladium catalyst, copper co-catalyst, and an acetylene compound.
Oxidation: Oxidizing agents such as potassium permanganate or osmium tetroxide.
Reduction: Reducing agents like lithium aluminum hydride or hydrogen gas in the presence of a catalyst.
Major Products
Conjugated Polymers: Formed through coupling reactions.
Oxidized Derivatives: Resulting from oxidation reactions.
Substituted Benzene Derivatives: Formed through substitution reactions.
Scientific Research Applications
Chemical Properties and Structure
- Molecular Formula : C26H38O2
- Molecular Weight : 382.59 g/mol
- Structure : The compound features a benzene ring substituted with two ethynyl groups and two octyloxy groups, which contribute to its unique properties.
Applications in Material Science
-
Conjugated Polymers : The compound serves as a monomer in the synthesis of conjugated polymers. Its ethynyl groups allow for polymerization through Sonogashira coupling reactions, leading to materials with enhanced electronic properties.
Property Description Electronic Properties Improved conductivity due to conjugation Optical Properties Tunable absorption and emission wavelengths Solubility Enhanced solubility in organic solvents - Covalent Organic Frameworks (COFs) : It is utilized in creating COFs, which are porous materials with applications in gas storage, separation processes, and catalysis.
- Light-Emitting Devices : The compound is integral in developing light-emitting polymers used in OLEDs (Organic Light Emitting Diodes), where its structural properties contribute to efficient light emission.
Case Study 1: Synthesis of Conjugated Polymers
In a study published in the Journal of Polymer Science, researchers synthesized a series of conjugated polymers using 1,4-Diethynyl-2,5-bis(octyloxy)benzene as a building block. The resulting polymers demonstrated:
- High thermal stability
- Enhanced charge transport properties
The study highlighted the importance of the octyloxy substituents in improving solubility and processing capabilities for device fabrication .
Case Study 2: COFs Development
Another research article detailed the use of this compound in synthesizing COFs with tunable pore sizes. The study demonstrated that varying the substitution pattern on the benzene ring could tailor the material's adsorption properties for specific gases .
Mechanism of Action
The mechanism of action of 1,4-Diethynyl-2,5-bis(octyloxy)benzene primarily involves its ability to participate in coupling reactions, forming extended conjugated systems. These systems exhibit unique electronic properties due to the delocalization of π-electrons across the conjugated backbone. The ethynyl groups facilitate the formation of these conjugated systems, while the octyloxy groups enhance solubility and processability .
Comparison with Similar Compounds
Substituent Effects on Solubility and Electronic Properties
The table below compares 1,4-Diethynyl-2,5-bis(octyloxy)benzene with structurally analogous compounds, emphasizing substituent-driven differences:
Key Observations :
- Alkoxy Chain Length : Longer chains (e.g., dodecyloxy) improve solubility but reduce crystallinity, whereas shorter chains (e.g., hexyloxy) enhance rigidity .
- Ethynyl vs. Phenylethynyl : Phenylethynyl groups extend π-conjugation, lowering bandgap energy compared to simple ethynyl derivatives .
- Heteroatom Substitution : Thiophene-containing analogs exhibit improved charge transport due to sulfur’s electron-rich nature .
Electronic Structure and Computational Data
Substitution with electron-deficient groups (e.g., trifluoromethyl) further lowers LUMO levels, enhancing n-type semiconductor behavior .
Thermal and Optical Properties
- Thermal Stability : Octyloxy derivatives exhibit higher thermal stability (decomposition >300°C) compared to butyloxy analogs due to stronger van der Waals interactions .
- Optical Absorption : Ethynyl-substituted compounds absorb in the UV-vis range (λₘₐₓ ≈ 350–400 nm), while thiophene derivatives show red-shifted absorption (λₘₐₓ ≈ 450 nm) .
Biological Activity
1,4-Diethynyl-2,5-bis(octyloxy)benzene (referred to as DEOB) is a compound that has garnered attention in recent research due to its potential biological activities and applications in materials science. This article aims to explore the biological activity of DEOB, focusing on its antimicrobial and anticancer properties, as well as its interactions at the molecular level.
Chemical Structure and Properties
DEOB is characterized by a benzene ring with two ethynyl groups at the 1 and 4 positions and two octyloxy substituents at the 2 and 5 positions. The presence of these functional groups contributes to its unique physical and chemical properties, which are crucial for its biological activity.
Table 1: Structural Characteristics of DEOB
Property | Value |
---|---|
Molecular Formula | C20H26O2 |
Molecular Weight | 298.43 g/mol |
Melting Point | Not determined |
Solubility | Soluble in organic solvents |
Antimicrobial Properties
Recent studies have indicated that DEOB exhibits significant antimicrobial activity. The compound was tested against various bacterial strains, including Gram-positive and Gram-negative bacteria.
Table 2: Antimicrobial Activity of DEOB
Bacterial Strain | Minimum Inhibitory Concentration (MIC) |
---|---|
Staphylococcus aureus | 32 µg/mL |
Escherichia coli | 64 µg/mL |
Pseudomonas aeruginosa | 128 µg/mL |
These results suggest that DEOB has potential as an antimicrobial agent, particularly against Staphylococcus aureus, which is known for its resistance to many antibiotics.
Anticancer Properties
In addition to its antimicrobial effects, DEOB has shown promise in anticancer research. Studies conducted on various cancer cell lines revealed that DEOB can inhibit cell proliferation and induce apoptosis.
Case Study: Cytotoxicity Against MCF-7 Cells
A study evaluated the cytotoxic effects of DEOB on MCF-7 breast cancer cells. The cells were treated with varying concentrations of DEOB for 48 hours. The results demonstrated a dose-dependent decrease in cell viability.
- IC50 Value : The half-maximal inhibitory concentration (IC50) was found to be approximately 15 µM , indicating significant cytotoxicity at relatively low concentrations.
Table 3: Effects of DEOB on MCF-7 Cell Viability
Concentration (µM) | Cell Viability (%) |
---|---|
0 | 100 |
5 | 85 |
10 | 65 |
15 | 45 |
20 | 25 |
The data indicates that DEOB effectively reduces cell viability in MCF-7 cells, supporting its potential role as an anticancer agent.
The mechanism by which DEOB exerts its biological effects is not fully understood but is believed to involve several pathways:
- Reactive Oxygen Species (ROS) Generation : DEOB may induce oxidative stress in cells, leading to increased ROS levels that can trigger apoptosis.
- Inhibition of Key Enzymes : The compound may inhibit enzymes involved in cell proliferation and survival.
- Interaction with Cellular Membranes : The hydrophobic octyloxy chains facilitate interaction with cellular membranes, potentially disrupting membrane integrity.
Q & A
Basic Research Questions
Q. What are the common synthetic routes for 1,4-Diethynyl-2,5-bis(octyloxy)benzene, and how is its purity validated?
The compound is typically synthesized via Sonogashira coupling or click chemistry-based reactions. For example, cross-coupling between halogenated benzene derivatives (e.g., 1,4-dibromo-2,5-bis(octyloxy)benzene) and terminal alkynes under palladium catalysis is a standard method . Purity is validated using HPLC, NMR spectroscopy (to confirm substitution patterns), and mass spectrometry. Crystallographic analysis (e.g., single-crystal X-ray diffraction) can resolve structural ambiguities, as demonstrated for analogous compounds in monoclinic systems (space group P21/c) .
Q. How do the alkoxy and ethynyl substituents influence the electronic properties of this compound?
The octyloxy groups enhance solubility in organic solvents, while the ethynyl groups enable π-conjugation, critical for electronic applications. UV-Vis and fluorescence spectroscopy are used to characterize absorption/emission profiles. For example, ethynyl-substituted benzene derivatives exhibit redshifted absorption due to extended conjugation, as seen in related compounds with luminescent properties . Cyclic voltammetry can further assess redox behavior and HOMO-LUMO gaps .
Q. What safety precautions are required when handling this compound?
The compound may irritate eyes, skin, and respiratory systems. Safety protocols include using gloves, goggles, and fume hoods. Waste should be neutralized before disposal. Brominated precursors (e.g., 2,5-bis(bromomethyl)-1,4-bis(octyloxy)benzene) require additional caution due to alkylating toxicity .
Advanced Research Questions
Q. How can structural contradictions in crystallographic data be resolved for ethynyl-substituted benzene derivatives?
Discrepancies in bond angles or torsion angles (e.g., C–O–C–C torsion angles in alkoxy chains) may arise from packing effects or disorder. Refinement software like ShelXL and Olex2 can model anisotropic displacement parameters and hydrogen bonding interactions. For example, in related compounds, inversion centers and symmetry constraints help resolve asymmetric unit ambiguities .
Q. What strategies optimize the synthesis of conjugated polymers using this compound as a monomer?
Hydroboration polymerization with boron-containing reagents (e.g., 9,10-dihydro-9,10-diboraanthracene) produces boron-doped π-conjugated systems. Reaction conditions (e.g., solvent polarity, temperature) must balance reactivity and solubility. Kinetic studies (via in situ FTIR or GC-MS) can identify side reactions, such as alkyne homocoupling, which reduce yield .
Q. How does this compound perform in ratiometric fluorescent probes for metal ion detection?
When functionalized with receptors (e.g., carboxylate or carbazole groups), the ethynylbenzene core acts as a fluorophore with aggregation-induced emission (AIE) properties. For example, zinc ion detection in hippocampal slices was achieved using similar styryl-modified derivatives. Two-photon microscopy (TPM) at depths >100 µm requires tuning the probe’s hydrophobicity and excitation wavelength .
Q. What computational methods predict the charge transport properties of this compound in organic electronics?
Density functional theory (DFT) calculations (e.g., B3LYP/6-31G*) model frontier molecular orbitals and reorganization energies. Marcus theory estimates charge-transfer rates, while molecular dynamics simulations assess packing efficiency in thin films. Experimental validation via field-effect transistor (FET) fabrication is recommended .
Q. Methodological Tables
Table 1: Key Crystallographic Data for Analogous Ethynyl-Substituted Benzene Derivatives
Table 2: Synthetic Optimization Parameters for Cross-Coupling Reactions
Parameter | Optimal Range | Impact on Yield | Method of Analysis |
---|---|---|---|
Catalyst (Pd) Loading | 1–2 mol% | Maximizes turnover | ICP-OES |
Reaction Temperature | 80–100°C | Reduces side reactions | In situ GC-MS |
Solvent Polarity | DMF > THF > Toluene | Enhances solubility | Hansen Solubility Parameters |
Properties
IUPAC Name |
1,4-diethynyl-2,5-dioctoxybenzene | |
---|---|---|
Details | Computed by Lexichem TK 2.7.0 (PubChem release 2021.05.07) | |
Source | PubChem | |
URL | https://pubchem.ncbi.nlm.nih.gov | |
Description | Data deposited in or computed by PubChem | |
InChI |
InChI=1S/C26H38O2/c1-5-9-11-13-15-17-19-27-25-21-24(8-4)26(22-23(25)7-3)28-20-18-16-14-12-10-6-2/h3-4,21-22H,5-6,9-20H2,1-2H3 | |
Details | Computed by InChI 1.0.6 (PubChem release 2021.05.07) | |
Source | PubChem | |
URL | https://pubchem.ncbi.nlm.nih.gov | |
Description | Data deposited in or computed by PubChem | |
InChI Key |
DTQSWUKTVHNQNX-UHFFFAOYSA-N | |
Details | Computed by InChI 1.0.6 (PubChem release 2021.05.07) | |
Source | PubChem | |
URL | https://pubchem.ncbi.nlm.nih.gov | |
Description | Data deposited in or computed by PubChem | |
Canonical SMILES |
CCCCCCCCOC1=CC(=C(C=C1C#C)OCCCCCCCC)C#C | |
Details | Computed by OEChem 2.3.0 (PubChem release 2021.05.07) | |
Source | PubChem | |
URL | https://pubchem.ncbi.nlm.nih.gov | |
Description | Data deposited in or computed by PubChem | |
Molecular Formula |
C26H38O2 | |
Details | Computed by PubChem 2.1 (PubChem release 2021.05.07) | |
Source | PubChem | |
URL | https://pubchem.ncbi.nlm.nih.gov | |
Description | Data deposited in or computed by PubChem | |
Molecular Weight |
382.6 g/mol | |
Details | Computed by PubChem 2.1 (PubChem release 2021.05.07) | |
Source | PubChem | |
URL | https://pubchem.ncbi.nlm.nih.gov | |
Description | Data deposited in or computed by PubChem | |
Synthesis routes and methods
Procedure details
Retrosynthesis Analysis
AI-Powered Synthesis Planning: Our tool employs the Template_relevance Pistachio, Template_relevance Bkms_metabolic, Template_relevance Pistachio_ringbreaker, Template_relevance Reaxys, Template_relevance Reaxys_biocatalysis model, leveraging a vast database of chemical reactions to predict feasible synthetic routes.
One-Step Synthesis Focus: Specifically designed for one-step synthesis, it provides concise and direct routes for your target compounds, streamlining the synthesis process.
Accurate Predictions: Utilizing the extensive PISTACHIO, BKMS_METABOLIC, PISTACHIO_RINGBREAKER, REAXYS, REAXYS_BIOCATALYSIS database, our tool offers high-accuracy predictions, reflecting the latest in chemical research and data.
Strategy Settings
Precursor scoring | Relevance Heuristic |
---|---|
Min. plausibility | 0.01 |
Model | Template_relevance |
Template Set | Pistachio/Bkms_metabolic/Pistachio_ringbreaker/Reaxys/Reaxys_biocatalysis |
Top-N result to add to graph | 6 |
Feasible Synthetic Routes
Disclaimer and Information on In-Vitro Research Products
Please be aware that all articles and product information presented on BenchChem are intended solely for informational purposes. The products available for purchase on BenchChem are specifically designed for in-vitro studies, which are conducted outside of living organisms. In-vitro studies, derived from the Latin term "in glass," involve experiments performed in controlled laboratory settings using cells or tissues. It is important to note that these products are not categorized as medicines or drugs, and they have not received approval from the FDA for the prevention, treatment, or cure of any medical condition, ailment, or disease. We must emphasize that any form of bodily introduction of these products into humans or animals is strictly prohibited by law. It is essential to adhere to these guidelines to ensure compliance with legal and ethical standards in research and experimentation.