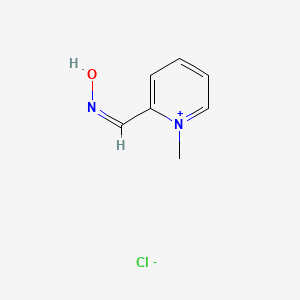
Pralidoxime chloride, (Z)-
Overview
Description
Pralidoxime chloride (2-PAM Cl), a quaternary pyridinium oxime, is a critical antidote for organophosphate (OP) poisoning and nerve agent exposure. Its chemical structure (C₇H₉ClN₂O) includes a positively charged pyridinium ring and an oxime group (-NOH) that reactivates acetylcholinesterase (AChE) inhibited by OP compounds . The (Z)-isomer (syn-configuration) is therapeutically active, enabling nucleophilic attack on phosphorylated AChE to restore enzyme function . Pralidoxime chloride is preferred over other salts (e.g., iodide, mesylate) due to its low molecular weight (172.6 Da), high water solubility, and rapid pharmacokinetics, achieving plasma concentrations of 7.5–9.9 µg/mL within 30–35 minutes post-intramuscular injection .
Preparation Methods
Synthetic Routes and Reaction Conditions
Pralidoxime chloride is synthesized by treating pyridine-2-carboxaldehyde with hydroxylamine, resulting in the formation of pyridine-2-aldoxime. This intermediate is then alkylated with methyl iodide to produce pralidoxime as the iodide salt . The iodide salt can be converted to the chloride salt through ion exchange processes.
Industrial Production Methods
In industrial settings, the preparation of pralidoxime chloride involves dissolving the compound in deionized water and methanol to create standard stock solutions. The solution is then subjected to various purification and quality control processes to ensure its efficacy and safety .
Chemical Reactions Analysis
Types of Reactions
Pralidoxime chloride undergoes several types of chemical reactions, including:
Substitution Reactions: The oxime group can participate in nucleophilic substitution reactions.
Oxidation and Reduction: The compound can undergo redox reactions, although these are less common in its typical applications.
Common Reagents and Conditions
Common reagents used in reactions involving pralidoxime chloride include hydroxylamine for the initial synthesis and methyl iodide for alkylation. Reaction conditions typically involve controlled temperatures and pH levels to ensure optimal yields .
Major Products Formed
The major product formed from the synthesis of pralidoxime chloride is the iodide salt, which can be further processed to obtain the chloride salt. Other by-products may include unreacted starting materials and minor impurities that are removed during purification .
Scientific Research Applications
Clinical Applications
1. Treatment of Organophosphate Poisoning
- Primary Use : Pralidoxime chloride is primarily used as an antidote in cases of organophosphate poisoning, which can occur from exposure to certain pesticides and nerve agents. It is often administered alongside atropine to counteract muscarinic symptoms .
- Case Studies : A randomized controlled trial involving 200 patients demonstrated that continuous infusion of pralidoxime resulted in lower mortality rates compared to bolus administration. Patients receiving a high-dose regimen showed significantly improved outcomes, including reduced need for intubation and mechanical ventilation .
2. Research on Efficacy
- Mortality Reduction : A study assessed the effectiveness of pralidoxime in reducing all-cause mortality among patients with acute organophosphorus insecticide poisoning. The results indicated a higher mortality rate in the pralidoxime group compared to placebo; however, significant variations were observed based on the type of organophosphate involved .
- Reactivation Studies : Research has shown that pralidoxime can variably reactivate butyrylcholinesterase (BuChE) in patients poisoned by specific diethyl organophosphates but was less effective against dimethyl compounds .
Industrial and Research Applications
1. Chemical Research
- Pralidoxime chloride is utilized in studies focused on enzyme reactivation mechanisms and the development of new antidotes for chemical warfare agents and pesticides. Its role in understanding cholinergic signaling pathways is critical for advancing therapeutic strategies against neurotoxins .
2. Development of Medical Countermeasures
- The compound is integral to creating autoinjectors designed for emergency use in cases of suspected nerve agent exposure. These devices are crucial for first responders and military personnel who may encounter chemical threats .
Pharmacokinetics
Pralidoxime chloride is characterized by its rapid distribution outside the central nervous system, with a significant volume of distribution and minimal protein binding. It is primarily excreted through urine, both as unchanged drug and metabolites, with a relatively short half-life that necessitates timely administration following exposure to organophosphates .
Summary Table of Key Findings
Application Area | Details |
---|---|
Primary Use | Antidote for organophosphate poisoning |
Mechanism | Reactivates acetylcholinesterase by cleaving phosphate-ester bonds |
Clinical Trials | Continuous infusion vs bolus injection; lower mortality with high-dose regimen |
Research Focus | Enzyme reactivation mechanisms; development of antidotes; safety protocols in industrial settings |
Pharmacokinetics | Rapidly distributed; minimal protein binding; excreted via urine |
Mechanism of Action
Pralidoxime chloride works by reactivating acetylcholinesterase, an enzyme that is inhibited by organophosphates. Organophosphates bind to the esteratic site of acetylcholinesterase, blocking its activity. Pralidoxime chloride binds to the anionic site of the enzyme and displaces the phosphate group from the serine residue, thereby regenerating the active enzyme. This process helps to restore normal neuromuscular function and alleviate the toxic effects of organophosphate poisoning .
Comparison with Similar Compounds
Comparison with Similar Oxime Compounds
Structural and Pharmacokinetic Differences
Key Findings :
- Potency : Pralidoxime chloride is 1.53× more potent than its iodide salt due to lower molecular weight .
- Reactivation Spectrum : Pralidoxime effectively reactivates diethyl OP-inhibited AChE (e.g., chlorpyrifos) but shows minimal activity against dimethyl OPs (e.g., malathion) . Obidoxime and HI-6 exhibit broader reactivation but carry higher toxicity risks .
- Stability : Pralidoxime chloride degrades in alkaline conditions (t½ < 24 hours at pH >9) but remains stable in autoinjectors for >2 years at 25–35°C . HI-6 and TMB-4 degrade faster in aqueous solutions .
A. Mortality Outcomes in OP Poisoning
dimethyl).
B. Adverse Effects
- Pralidoxime chloride causes transient hypertension, dizziness, and blurred vision but is well-tolerated compared to obidoxime (hepatotoxicity) and HI-6 (cardiotoxicity).
- Accidental autoinjector injections in non-poisoned individuals result in mild, self-limiting symptoms (e.g., nausea).
Stability and Formulation Challenges
Pharmacokinetic Profile
Parameter | Pralidoxime Chloride | Obidoxime |
---|---|---|
Bioavailability | ~90% (IM) | ~75% (oral) |
Volume of Distribution | 0.6–2.7 L/kg | 0.3–0.5 L/kg |
Elimination | Renal excretion (unchanged) | Hepatic metabolism |
Biological Activity
Pralidoxime chloride (Z), an oxime compound, plays a critical role in the reactivation of acetylcholinesterase (AChE), particularly after inhibition by organophosphate compounds. This article reviews its biological activity, mechanisms of action, pharmacokinetics, and clinical efficacy based on diverse research findings.
Target Enzyme:
Pralidoxime chloride primarily targets acetylcholinesterase, an enzyme essential for hydrolyzing acetylcholine in the synaptic cleft. Inhibition of AChE leads to an accumulation of acetylcholine, resulting in overstimulation of cholinergic receptors, which can be life-threatening.
Mode of Action:
Organophosphates bind to the esteratic site of AChE, leading to its inactivation. Pralidoxime chloride acts by binding to the anionic site of AChE, allowing it to displace the organophosphate and reactivating the enzyme. This mechanism is crucial for restoring normal neurotransmission following organophosphate poisoning .
Biochemical Pathways:
The primary biochemical pathway affected by pralidoxime chloride is the cholinergic synapse. Its effectiveness is contingent upon timely administration, ideally within 24 hours post-exposure to organophosphates.
Pharmacokinetics
Pralidoxime chloride is distributed primarily outside the central nervous system and is rapidly excreted via urine. The drug undergoes hepatic metabolism and does not bind significantly to plasma proteins . Its half-life and pharmacokinetic profile are critical for determining dosing regimens in clinical settings.
Case Studies and Trials
-
Randomized Controlled Trials:
A significant study conducted in Sri Lanka assessed the impact of pralidoxime chloride on mortality rates in patients with organophosphate poisoning. In this trial, 235 patients were randomized to receive either pralidoxime or a saline placebo. The results indicated that pralidoxime produced substantial reactivation of red cell AChE but did not significantly reduce mortality (24.8% vs. 15.8% in placebo) . -
Dosage and Timing:
The study highlighted that the timing of administration greatly influences outcomes. Patients treated within two hours post-exposure had better survival rates compared to those treated later . Optimal dosages included a loading dose of 2 g followed by continuous infusion . -
Comparative Efficacy:
In vitro studies have shown that pralidoxime chloride effectively reactivates AChE inhibited by various organophosphate compounds, with varying efficacy depending on the specific agent involved . For instance, it was found to be effective against diethyl and dimethyl organophosphates but less so against more complex structures.
Reactivation Efficiency
The reactivation efficiency of pralidoxime varies with different organophosphate agents. For example:
Organophosphate | Reactivation Efficacy |
---|---|
Paraoxon | High |
Chlorpyrifos | Moderate |
Methamidophos | Variable |
Studies indicate that pralidoxime's effectiveness can be influenced by factors such as concentration and the specific type of organophosphate involved .
Q & A
Basic Research Questions
Q. What is the mechanism of action of pralidoxime chloride in reactivating acetylcholinesterase (AChE) inhibited by organophosphates?
Pralidoxime chloride reactivates AChE by nucleophilic attack on the phosphorylated serine residue, displacing the organophosphate moiety. Its quaternary ammonium group facilitates binding to the anionic site of AChE, while the oxime group regenerates the enzyme’s active site. This mechanism is pH-dependent, with optimal activity at neutral to slightly alkaline conditions. Researchers should validate reactivation efficiency using in vitro assays (e.g., Ellman’s method) and monitor reactivation kinetics under varying pH and temperature conditions .
Q. What analytical methods are recommended for quantifying pralidoxime chloride in biological samples?
Reverse-phase high-performance liquid chromatography (RP-HPLC) with UV detection at 270–293 nm is standard. For highly polar pralidoxime, ion-pairing reagents (e.g., tetraethylammonium chloride, sodium octanesulfonate) are added to the mobile phase to improve retention. Chaotropic salt-enhanced chromatography (e.g., 0.1 M perchlorate) has shown superior resolution for stability studies. Method validation should include linearity (5–50 µg/mL), recovery (>99%), and precision (RSD <1%) .
Q. What dosing strategies optimize pralidoxime chloride efficacy in organophosphate poisoning?
Clinical studies recommend a 30 mg/kg bolus followed by 8 mg/kg/h infusion to maintain plasma levels >4 µg/mL, the threshold for AChE reactivation. Intramuscular administration (e.g., auto-injectors) is used when IV access is unavailable. Researchers should design dose-response trials with stratified subgroups (e.g., renal impairment, pesticide type) and monitor neuromuscular recovery and plasma cholinesterase activity .
Q. How does pralidoxime chloride’s pharmacokinetic profile influence its therapeutic window?
Pralidoxime has a short half-life (~2 hours) and volume of distribution (0.6–2.7 L/kg), necessitating repeated dosing. Renal excretion accounts for 80–90% of clearance, with tubular secretion mediated by organic cation transporters. Researchers should model pharmacokinetics using non-linear mixed-effects software (e.g., NONMEM) to optimize infusion regimens in renal-impaired patients .
Advanced Research Questions
Q. How can contradictory clinical efficacy data for pralidoxime chloride be reconciled?
Disparate outcomes arise from delayed administration (>36 hours post-exposure), aging of phosphorylated AChE, and variability in organophosphate toxicity (e.g., dimethyl vs. diethyl compounds). Advanced studies should incorporate biomarkers (e.g., RBC AChE activity) and in silico models to predict reactivation potential. The WHO-recommended regimen lacks universal efficacy, particularly for lipophilic nerve agents (e.g., soman), necessitating adjunct therapies like bioscavengers .
Q. What mechanisms underlie pralidoxime chloride’s renal tubular secretion?
Pralidoxime is secreted via organic cation transporters (OCT2), competing with endogenous bases (e.g., thiamine). Studies using probenecid or cimetidine (OCT inhibitors) show reduced clearance. Acidic urine pH enhances reabsorption, while alkaline pH increases ionized fractions. Researchers should use renal micropuncture or transfected cell models to map transporter interactions .
Q. How can pharmacokinetic-pharmacodynamic (PK/PD) modeling improve dosing in acute poisoning?
Mechanistic PK/PD models integrate AChE reactivation rates, organophosphate re-inhibition, and aging kinetics. For example, continuous IV infusion (0.5 g/h) sustains therapeutic levels better than bolus dosing. Researchers should validate models with ex vivo AChE activity measurements and Bayesian forecasting for real-time dose adjustments .
Q. What factors influence pralidoxime chloride’s stability in auto-injectors under field conditions?
Degradation is accelerated by alkaline pH (>7.5), heat (>25°C), and hydroxyl ions, forming dehydration products (e.g., nitriles). Stability studies using accelerated aging (40°C/75% RH) and UHPLC-UV/MS detect impurities (e.g., <0.05% by area normalization). Researchers should optimize auto-injector formulations with buffering agents (pH 3.5–4.5) and lyophilization to enhance shelf life .
Q. How does pralidoxime chloride interact with atropine in combined antidotal therapy?
Atropine antagonizes muscarinic effects (e.g., bronchospasm), while pralidoxime reverses nicotinic paralysis. Synergy studies in animal models show reduced mortality when both are administered within 10 minutes of exposure. Researchers should use isobolographic analysis to quantify interaction magnitudes and assess risks (e.g., hypertension from excessive pralidoxime) in geriatric or hypertensive cohorts .
Q. What methodologies are used for impurity profiling in pralidoxime chloride formulations?
USP monographs specify RP-HPLC with C18 columns and gradient elution (83:17 water:acetonitrile) to resolve anti-isomers (relative retention 0.9) and degradation products. Forced degradation studies (acid/alkali hydrolysis, oxidation) identify major impurities. Quantitation limits (0.05%) require validation per ICH Q3B guidelines, with reference standards cross-checked via NMR and HRMS .
Properties
IUPAC Name |
(NZ)-N-[(1-methylpyridin-1-ium-2-yl)methylidene]hydroxylamine;chloride | |
---|---|---|
Details | Computed by Lexichem TK 2.7.0 (PubChem release 2021.05.07) | |
Source | PubChem | |
URL | https://pubchem.ncbi.nlm.nih.gov | |
Description | Data deposited in or computed by PubChem | |
InChI |
InChI=1S/C7H8N2O.ClH/c1-9-5-3-2-4-7(9)6-8-10;/h2-6H,1H3;1H | |
Details | Computed by InChI 1.0.6 (PubChem release 2021.05.07) | |
Source | PubChem | |
URL | https://pubchem.ncbi.nlm.nih.gov | |
Description | Data deposited in or computed by PubChem | |
InChI Key |
HIGSLXSBYYMVKI-UHFFFAOYSA-N | |
Details | Computed by InChI 1.0.6 (PubChem release 2021.05.07) | |
Source | PubChem | |
URL | https://pubchem.ncbi.nlm.nih.gov | |
Description | Data deposited in or computed by PubChem | |
Canonical SMILES |
C[N+]1=CC=CC=C1C=NO.[Cl-] | |
Details | Computed by OEChem 2.3.0 (PubChem release 2021.05.07) | |
Source | PubChem | |
URL | https://pubchem.ncbi.nlm.nih.gov | |
Description | Data deposited in or computed by PubChem | |
Isomeric SMILES |
C[N+]1=CC=CC=C1/C=N\O.[Cl-] | |
Details | Computed by OEChem 2.3.0 (PubChem release 2021.05.07) | |
Source | PubChem | |
URL | https://pubchem.ncbi.nlm.nih.gov | |
Description | Data deposited in or computed by PubChem | |
Molecular Formula |
C7H9ClN2O | |
Details | Computed by PubChem 2.1 (PubChem release 2021.05.07) | |
Source | PubChem | |
URL | https://pubchem.ncbi.nlm.nih.gov | |
Description | Data deposited in or computed by PubChem | |
Molecular Weight |
172.61 g/mol | |
Details | Computed by PubChem 2.1 (PubChem release 2021.05.07) | |
Source | PubChem | |
URL | https://pubchem.ncbi.nlm.nih.gov | |
Description | Data deposited in or computed by PubChem | |
Retrosynthesis Analysis
AI-Powered Synthesis Planning: Our tool employs the Template_relevance Pistachio, Template_relevance Bkms_metabolic, Template_relevance Pistachio_ringbreaker, Template_relevance Reaxys, Template_relevance Reaxys_biocatalysis model, leveraging a vast database of chemical reactions to predict feasible synthetic routes.
One-Step Synthesis Focus: Specifically designed for one-step synthesis, it provides concise and direct routes for your target compounds, streamlining the synthesis process.
Accurate Predictions: Utilizing the extensive PISTACHIO, BKMS_METABOLIC, PISTACHIO_RINGBREAKER, REAXYS, REAXYS_BIOCATALYSIS database, our tool offers high-accuracy predictions, reflecting the latest in chemical research and data.
Strategy Settings
Precursor scoring | Relevance Heuristic |
---|---|
Min. plausibility | 0.01 |
Model | Template_relevance |
Template Set | Pistachio/Bkms_metabolic/Pistachio_ringbreaker/Reaxys/Reaxys_biocatalysis |
Top-N result to add to graph | 6 |
Feasible Synthetic Routes
Disclaimer and Information on In-Vitro Research Products
Please be aware that all articles and product information presented on BenchChem are intended solely for informational purposes. The products available for purchase on BenchChem are specifically designed for in-vitro studies, which are conducted outside of living organisms. In-vitro studies, derived from the Latin term "in glass," involve experiments performed in controlled laboratory settings using cells or tissues. It is important to note that these products are not categorized as medicines or drugs, and they have not received approval from the FDA for the prevention, treatment, or cure of any medical condition, ailment, or disease. We must emphasize that any form of bodily introduction of these products into humans or animals is strictly prohibited by law. It is essential to adhere to these guidelines to ensure compliance with legal and ethical standards in research and experimentation.