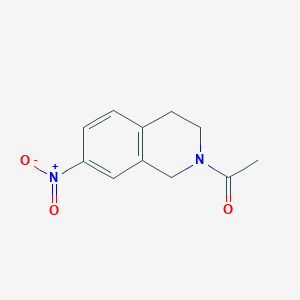
1-(7-Nitro-3,4-dihydroisoquinolin-2(1H)-yl)ethanone
Overview
Description
1-(7-Nitro-3,4-dihydroisoquinolin-2(1H)-yl)ethanone is a tetrahydroisoquinoline derivative characterized by a nitro (-NO₂) substituent at position 7 of the isoquinoline ring and an ethanone group at position 2. The core structure consists of a partially saturated isoquinoline scaffold, which is widely studied for its biological relevance. Tetrahydroisoquinoline derivatives exhibit diverse pharmacological activities, including neurotoxicity, antitumor, antimicrobial, and central nervous system (CNS) receptor modulation . The nitro group at position 7 enhances electron-withdrawing effects, influencing both chemical reactivity and biological activity. This compound serves as a critical intermediate in synthesizing bioactive molecules, particularly in medicinal chemistry for drug discovery .
Preparation Methods
Synthetic Routes and Reaction Conditions
The synthesis of 1-(7-Nitro-3,4-dihydroisoquinolin-2(1H)-yl)ethanone typically involves the nitration of 3,4-dihydroisoquinoline followed by the introduction of the ethanone group. One common method involves the use of nitric acid and sulfuric acid to nitrate 3,4-dihydroisoquinoline, forming 7-nitro-3,4-dihydroisoquinoline. This intermediate is then reacted with ethanoyl chloride in the presence of a base such as pyridine to yield the final product .
Industrial Production Methods
Industrial production of this compound may involve similar synthetic routes but on a larger scale. The use of continuous flow reactors and automated systems can enhance the efficiency and yield of the production process. Additionally, the purification of the final product is achieved through techniques such as recrystallization and chromatography.
Chemical Reactions Analysis
Types of Reactions
1-(7-Nitro-3,4-dihydroisoquinolin-2(1H)-yl)ethanone undergoes various chemical reactions, including:
Oxidation: The compound can be oxidized to form corresponding nitroisoquinoline derivatives.
Reduction: The nitro group can be reduced to an amino group using reducing agents such as hydrogen gas in the presence of a palladium catalyst.
Substitution: The ethanone group can undergo nucleophilic substitution reactions with various nucleophiles.
Common Reagents and Conditions
Oxidation: Potassium permanganate or chromium trioxide in acidic conditions.
Reduction: Hydrogen gas with palladium on carbon (Pd/C) or sodium borohydride.
Substitution: Nucleophiles such as amines or thiols in the presence of a base.
Major Products Formed
Oxidation: Nitroisoquinoline derivatives.
Reduction: Aminoisoquinoline derivatives.
Substitution: Substituted ethanone derivatives.
Scientific Research Applications
1-(7-Nitro-3,4-dihydroisoquinolin-2(1H)-yl)ethanone has various applications in scientific research:
Chemistry: Used as a building block for the synthesis of more complex isoquinoline derivatives.
Biology: Studied for its potential biological activities, including antimicrobial and anticancer properties.
Medicine: Investigated for its potential use in drug development, particularly in the design of novel therapeutic agents.
Industry: Utilized in the production of dyes, pigments, and other industrial chemicals.
Mechanism of Action
The mechanism of action of 1-(7-Nitro-3,4-dihydroisoquinolin-2(1H)-yl)ethanone involves its interaction with specific molecular targets and pathways. The nitro group can undergo bioreduction to form reactive intermediates that can interact with cellular components, leading to various biological effects. The compound may also inhibit specific enzymes or receptors, contributing to its observed biological activities.
Comparison with Similar Compounds
Comparison with Structurally Similar Compounds
Structural Variations and Key Properties
The following table summarizes structural analogs of 1-(7-nitro-3,4-dihydroisoquinolin-2(1H)-yl)ethanone, highlighting substituents, molecular properties, and applications:
Detailed Analysis of Structural and Functional Differences
Nitro vs. Halogen Substituents
- Nitro Group (NO₂): The nitro group at position 7 (target compound) enhances electrophilic reactivity, making it suitable for reduction to amines (e.g., for generating bioactive amino derivatives).
- The 7-bromo-6-nitro derivative () demonstrates how halogen-nitro combinations can stabilize molecular interactions in enzyme inhibition .
Ethanone Modifications
- Trifluoroethanone (CF₃): Compounds with trifluoroethanone () exhibit enhanced metabolic stability due to the electron-withdrawing CF₃ group, which resists oxidative degradation. This modification is critical in developing long-acting inhibitors .
- Unmodified Ethanone: The parent structure () serves as a versatile intermediate for introducing diverse substituents via acylations or nucleophilic substitutions .
Amino Substituents (NH₂)
- The 7-amino derivative () is pivotal in designing CD44 antagonists and kinase inhibitors. Amino groups facilitate hydrogen bonding, improving target binding affinity .
Biological Activity
1-(7-Nitro-3,4-dihydroisoquinolin-2(1H)-yl)ethanone, also known as 1-(3,4-dihydro-7-nitro-2(1H)-isoquinolinyl)ethanone, is a compound of significant interest due to its potential biological activities. This article reviews its biological properties, synthesis methods, and applications in medicinal chemistry.
- Molecular Formula : C11H12N2O3
- Molecular Weight : 220.22 g/mol
- CAS Number : 99365-63-6
The compound features a nitro group at the 7th position and an ethanone group at the 1st position of the isoquinoline ring, which contributes to its unique chemical reactivity and biological activity .
Synthesis Methods
This compound can be synthesized through the nitration of 3,4-dihydroisoquinoline followed by acetylation. The general steps include:
- Nitration : Using a mixture of nitric acid and sulfuric acid to introduce the nitro group.
- Acetylation : Reacting the nitrated intermediate with ethanoyl chloride in the presence of a base like pyridine.
This method allows for efficient production of the compound in a laboratory setting .
Antimicrobial Properties
Research indicates that compounds with similar structures to this compound exhibit antimicrobial activity. A study on related isoquinoline derivatives showed effectiveness against various bacterial strains, suggesting potential applications in treating infections .
Anticancer Activity
The compound has been investigated for its anticancer properties. In vitro studies have demonstrated that it can induce apoptosis in cancer cells through mechanisms involving reactive oxygen species (ROS) generation and mitochondrial dysfunction .
Neuroprotective Effects
There is emerging evidence that compounds within the isoquinoline family may possess neuroprotective effects. They could modulate neurotransmitter systems and exhibit potential in treating neurodegenerative diseases .
The biological activity of this compound is believed to involve:
- Bioreduction : The nitro group can be reduced to form reactive intermediates that interact with cellular components.
- Enzyme Inhibition : The compound may inhibit specific enzymes or receptors involved in disease pathways.
These interactions contribute to its observed therapeutic effects .
Comparative Analysis with Similar Compounds
Compound Name | Structure | Notable Activity |
---|---|---|
7-Nitro-3,4-dihydroisoquinoline | Structure | Antimicrobial |
2-Benzyl-7-nitro-3,4-dihydroisoquinoline | Structure | Anticancer |
tert-Butyl 7-nitro-3,4-dihydroisoquinoline-2(1H)-carboxylate | Structure | Neuroprotective |
Case Studies
- Antimicrobial Efficacy : A study published in Phytochemistry demonstrated that derivatives of isoquinoline exhibited significant antibacterial activity against Staphylococcus aureus and Escherichia coli, with minimum inhibitory concentrations (MICs) comparable to standard antibiotics .
- Apoptosis Induction in Cancer Cells : Research conducted on lung cancer cell lines showed that treatment with isoquinoline derivatives led to increased apoptosis markers and decreased cell viability, indicating potential for drug development in oncology .
Q & A
Q. What are the established synthetic routes for preparing 1-(7-Nitro-3,4-dihydroisoquinolin-2(1H)-yl)ethanone and its derivatives?
Basic Research Question
The synthesis of tetrahydroisoquinoline derivatives often involves acetylation or cyclization reactions. A representative method includes reacting a substituted tetrahydroisoquinoline core with acetylating agents like acetic anhydride in pyridine under argon, yielding derivatives with high purity (89% yield in one protocol) . Alternative routes employ silica-supported polyphosphoric acid (PPA) to facilitate cyclization, achieving efficient ring closure and functionalization . For nitro-substituted derivatives, nitration of precursor aromatic rings may precede acetylation, requiring careful control of reaction conditions to avoid over-oxidation.
Q. How is structural elucidation of this compound achieved using spectroscopic techniques?
Basic Research Question
Structural confirmation relies on NMR (¹H, ¹³C), IR, and HRMS data. For example:
- ¹H NMR : Signals at δ 1.66 (s, 3H) confirm the acetyl group, while aromatic protons (δ 6.47–6.82) and nitro-substituent effects are observed in splitting patterns .
- ¹³C NMR : Carbonyl resonance near δ 170.0 and aromatic carbons (δ 120–150) validate the core structure .
- HRMS : Exact mass matching (e.g., [M+H]+ calculated 390.20637 vs. observed 390.20671) ensures molecular integrity .
Q. What biological screening approaches are used to evaluate the bioactivity of nitro-substituted tetrahydroisoquinoline derivatives?
Basic Research Question
Initial screening often involves enzyme inhibition assays. For HIV-1 reverse transcriptase (RT), compounds are tested at 100 µM concentrations, with inhibition percentages calculated relative to controls. For example, derivatives with electron-withdrawing nitro groups may show altered potency compared to methoxy-substituted analogs (e.g., 74.82% inhibition for methoxy derivatives vs. pending nitro-substituted data) . Cell-based assays (e.g., HEK293 models) are used to assess functional activity, such as calcium channel blockade or receptor modulation .
Q. How do structure-activity relationship (SAR) studies guide the optimization of tetrahydroisoquinoline derivatives for enhanced bioactivity?
Advanced Research Question
SAR studies focus on substituent effects:
- Electron-withdrawing groups (e.g., NO₂) : May enhance binding to hydrophobic pockets in targets like HIV-1 RT or CD44, as seen in analogs with chloro or nitro substituents .
- Positional effects : Para-substitutions on aromatic rings often improve potency compared to ortho/meta positions. For example, para-chloro in HIV-1 RT inhibitors increased inhibition by ~20% .
- Conformational flexibility : Bulky substituents (e.g., allyl groups) can reduce activity by steric hindrance, as observed in lower yields (48%) for allylated derivatives .
Q. What computational strategies are employed to predict the mechanism of action of nitro-substituted tetrahydroisoquinoline derivatives?
Advanced Research Question
Molecular docking and free-energy calculations are used to model interactions with targets like CD44 or HIV-1 RT. For CD44 antagonists, compounds such as Can159 showed high theoretical affinity (ΔG = −8.2 kcal/mol) by stabilizing CD44’s hyaluronic acid-binding domain via hydrophobic and hydrogen-bond interactions . Docking into the HIV-1 RT non-nucleoside inhibitor binding pocket (NNIBP) revealed critical contacts with residues Tyr181 and Trp229, correlating with experimental IC₅₀ values .
Q. How can researchers address discrepancies in reported substituent effects on biological activity?
Advanced Research Question
Contradictions (e.g., nitro vs. methoxy group efficacy) require systematic validation:
- Assay standardization : Differences in inhibition percentages (e.g., 74.82% vs. 72.58% for similar compounds) may arise from variations in enzyme sources or buffer conditions .
- Computational validation : Density Functional Theory (DFT) calculations can compare electronic profiles (e.g., nitro’s electron-withdrawing nature vs. methoxy’s electron-donating effects) to rationalize activity trends .
- Synthetic reproducibility : Replicating protocols (e.g., silica-PPA cyclization vs. acetic anhydride acetylation ) ensures substituent effects are not confounded by synthetic artifacts.
Q. What are the key considerations for designing derivatives with improved metabolic stability and selectivity?
Advanced Research Question
- Lipophilicity reduction : Replacing lipophilic groups (e.g., benzyl) with polar substituents (e.g., hydroxypropyl) minimizes CYP450 inhibition, as demonstrated in N-type calcium channel blockers .
- Pro-drug strategies : Ester or amide prodrugs of 7-nitro derivatives could enhance bioavailability, leveraging successful precedents in HIV-1 RT inhibitor design .
- Isosteric replacements : Bioisosteres like tetrazoles (e.g., Can159 ) maintain target affinity while improving solubility.
Properties
IUPAC Name |
1-(7-nitro-3,4-dihydro-1H-isoquinolin-2-yl)ethanone | |
---|---|---|
Details | Computed by LexiChem 2.6.6 (PubChem release 2019.06.18) | |
Source | PubChem | |
URL | https://pubchem.ncbi.nlm.nih.gov | |
Description | Data deposited in or computed by PubChem | |
InChI |
InChI=1S/C11H12N2O3/c1-8(14)12-5-4-9-2-3-11(13(15)16)6-10(9)7-12/h2-3,6H,4-5,7H2,1H3 | |
Details | Computed by InChI 1.0.5 (PubChem release 2019.06.18) | |
Source | PubChem | |
URL | https://pubchem.ncbi.nlm.nih.gov | |
Description | Data deposited in or computed by PubChem | |
InChI Key |
BKJRHIYYEKJYTP-UHFFFAOYSA-N | |
Details | Computed by InChI 1.0.5 (PubChem release 2019.06.18) | |
Source | PubChem | |
URL | https://pubchem.ncbi.nlm.nih.gov | |
Description | Data deposited in or computed by PubChem | |
Canonical SMILES |
CC(=O)N1CCC2=C(C1)C=C(C=C2)[N+](=O)[O-] | |
Details | Computed by OEChem 2.1.5 (PubChem release 2019.06.18) | |
Source | PubChem | |
URL | https://pubchem.ncbi.nlm.nih.gov | |
Description | Data deposited in or computed by PubChem | |
Molecular Formula |
C11H12N2O3 | |
Details | Computed by PubChem 2.1 (PubChem release 2019.06.18) | |
Source | PubChem | |
URL | https://pubchem.ncbi.nlm.nih.gov | |
Description | Data deposited in or computed by PubChem | |
Molecular Weight |
220.22 g/mol | |
Details | Computed by PubChem 2.1 (PubChem release 2021.05.07) | |
Source | PubChem | |
URL | https://pubchem.ncbi.nlm.nih.gov | |
Description | Data deposited in or computed by PubChem | |
Retrosynthesis Analysis
AI-Powered Synthesis Planning: Our tool employs the Template_relevance Pistachio, Template_relevance Bkms_metabolic, Template_relevance Pistachio_ringbreaker, Template_relevance Reaxys, Template_relevance Reaxys_biocatalysis model, leveraging a vast database of chemical reactions to predict feasible synthetic routes.
One-Step Synthesis Focus: Specifically designed for one-step synthesis, it provides concise and direct routes for your target compounds, streamlining the synthesis process.
Accurate Predictions: Utilizing the extensive PISTACHIO, BKMS_METABOLIC, PISTACHIO_RINGBREAKER, REAXYS, REAXYS_BIOCATALYSIS database, our tool offers high-accuracy predictions, reflecting the latest in chemical research and data.
Strategy Settings
Precursor scoring | Relevance Heuristic |
---|---|
Min. plausibility | 0.01 |
Model | Template_relevance |
Template Set | Pistachio/Bkms_metabolic/Pistachio_ringbreaker/Reaxys/Reaxys_biocatalysis |
Top-N result to add to graph | 6 |
Feasible Synthetic Routes
Disclaimer and Information on In-Vitro Research Products
Please be aware that all articles and product information presented on BenchChem are intended solely for informational purposes. The products available for purchase on BenchChem are specifically designed for in-vitro studies, which are conducted outside of living organisms. In-vitro studies, derived from the Latin term "in glass," involve experiments performed in controlled laboratory settings using cells or tissues. It is important to note that these products are not categorized as medicines or drugs, and they have not received approval from the FDA for the prevention, treatment, or cure of any medical condition, ailment, or disease. We must emphasize that any form of bodily introduction of these products into humans or animals is strictly prohibited by law. It is essential to adhere to these guidelines to ensure compliance with legal and ethical standards in research and experimentation.