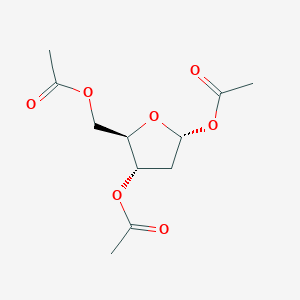
1,3,5-tri-O-Acetyl-2-Deoxy-alpha-D-Erythro-Pentofuranose
Overview
Description
1,3,5-Tri-O-Acetyl-2-Deoxy-alpha-D-Erythro-Pentofuranose is a synthetic derivative of pentofuranose, a five-membered ring sugar molecule. This compound is characterized by the presence of three acetyl groups attached to the oxygen atoms at the 1, 3, and 5 positions of the furanose ring, and a deoxy group at the 2 position. It is a versatile intermediate in organic synthesis and has applications in various scientific fields.
Synthetic Routes and Reaction Conditions:
Acetylation of Pentofuranose: The compound can be synthesized by acetylating pentofuranose using acetic anhydride in the presence of a catalyst such as pyridine or DMAP (4-Dimethylaminopyridine). The reaction is typically carried out at elevated temperatures to ensure complete acetylation.
Deoxygenation: The deoxy group at the 2 position can be introduced by treating the pentofuranose with a deoxygenating agent such as triphenylphosphine and carbon tetrachloride.
Industrial Production Methods:
Batch Process: In an industrial setting, the compound is often produced using a batch process where the reactants are mixed in a reactor, heated, and stirred to ensure uniform reaction. The product is then purified through crystallization or chromatography.
Continuous Flow Process: For large-scale production, a continuous flow process can be employed where the reactants are continuously fed into a reactor, and the product is continuously extracted and purified.
Types of Reactions:
Oxidation: The compound can undergo oxidation reactions to form various oxidized derivatives. Common oxidizing agents include hydrogen peroxide, sodium periodate, and TEMPO (2,2,6,6-Tetramethylpiperidine-1-oxyl).
Reduction: Reduction reactions can be performed using reducing agents such as lithium aluminum hydride (LAH) or sodium borohydride to produce reduced derivatives.
Substitution: Substitution reactions can be carried out by treating the compound with nucleophiles such as amines, alcohols, or halides.
Common Reagents and Conditions:
Oxidation: Hydrogen peroxide (H2O2), sodium periodate (NaIO4), TEMPO, aqueous conditions.
Reduction: Lithium aluminum hydride (LiAlH4), sodium borohydride (NaBH4), ether or THF (tetrahydrofuran) solvents.
Substitution: Amines, alcohols, halides, polar aprotic solvents.
Major Products Formed:
Oxidation Products: Carboxylic acids, aldehydes, ketones.
Reduction Products: Alcohols, aldehydes.
Substitution Products: Amides, ethers, halides.
Scientific Research Applications
Chemistry: The compound is used as an intermediate in the synthesis of various organic molecules, including pharmaceuticals and agrochemicals.
Biology: It serves as a building block for the synthesis of nucleosides and nucleotides, which are essential for DNA and RNA research.
Medicine: The compound is utilized in the development of antiviral and anticancer drugs due to its ability to interfere with nucleic acid synthesis.
Industry: It is employed in the production of biodegradable polymers and other materials with specific chemical properties.
Mechanism of Action
The compound exerts its effects through its ability to interact with nucleic acids and enzymes involved in nucleotide metabolism. It can inhibit enzymes such as DNA polymerases and RNA polymerases, leading to the disruption of nucleic acid synthesis. The molecular targets include the active sites of these enzymes, where the compound binds and inhibits their activity.
Comparison with Similar Compounds
2-Deoxyribose
2-Deoxy-D-erythro-pentofuranose
1,3,5-Tri-O-methyl-2-deoxy-alpha-D-erythro-pentofuranose
Properties
IUPAC Name |
[(2R,3S,5R)-3,5-diacetyloxyoxolan-2-yl]methyl acetate | |
---|---|---|
Details | Computed by LexiChem 2.6.6 (PubChem release 2019.06.18) | |
Source | PubChem | |
URL | https://pubchem.ncbi.nlm.nih.gov | |
Description | Data deposited in or computed by PubChem | |
InChI |
InChI=1S/C11H16O7/c1-6(12)15-5-10-9(16-7(2)13)4-11(18-10)17-8(3)14/h9-11H,4-5H2,1-3H3/t9-,10+,11-/m0/s1 | |
Details | Computed by InChI 1.0.5 (PubChem release 2019.06.18) | |
Source | PubChem | |
URL | https://pubchem.ncbi.nlm.nih.gov | |
Description | Data deposited in or computed by PubChem | |
InChI Key |
QAGMBTAACMQRSS-AXFHLTTASA-N | |
Details | Computed by InChI 1.0.5 (PubChem release 2019.06.18) | |
Source | PubChem | |
URL | https://pubchem.ncbi.nlm.nih.gov | |
Description | Data deposited in or computed by PubChem | |
Canonical SMILES |
CC(=O)OCC1C(CC(O1)OC(=O)C)OC(=O)C | |
Details | Computed by OEChem 2.1.5 (PubChem release 2019.06.18) | |
Source | PubChem | |
URL | https://pubchem.ncbi.nlm.nih.gov | |
Description | Data deposited in or computed by PubChem | |
Isomeric SMILES |
CC(=O)OC[C@@H]1[C@H](C[C@H](O1)OC(=O)C)OC(=O)C | |
Details | Computed by OEChem 2.1.5 (PubChem release 2019.06.18) | |
Source | PubChem | |
URL | https://pubchem.ncbi.nlm.nih.gov | |
Description | Data deposited in or computed by PubChem | |
Molecular Formula |
C11H16O7 | |
Details | Computed by PubChem 2.1 (PubChem release 2019.06.18) | |
Source | PubChem | |
URL | https://pubchem.ncbi.nlm.nih.gov | |
Description | Data deposited in or computed by PubChem | |
Molecular Weight |
260.24 g/mol | |
Details | Computed by PubChem 2.1 (PubChem release 2021.05.07) | |
Source | PubChem | |
URL | https://pubchem.ncbi.nlm.nih.gov | |
Description | Data deposited in or computed by PubChem | |
Retrosynthesis Analysis
AI-Powered Synthesis Planning: Our tool employs the Template_relevance Pistachio, Template_relevance Bkms_metabolic, Template_relevance Pistachio_ringbreaker, Template_relevance Reaxys, Template_relevance Reaxys_biocatalysis model, leveraging a vast database of chemical reactions to predict feasible synthetic routes.
One-Step Synthesis Focus: Specifically designed for one-step synthesis, it provides concise and direct routes for your target compounds, streamlining the synthesis process.
Accurate Predictions: Utilizing the extensive PISTACHIO, BKMS_METABOLIC, PISTACHIO_RINGBREAKER, REAXYS, REAXYS_BIOCATALYSIS database, our tool offers high-accuracy predictions, reflecting the latest in chemical research and data.
Strategy Settings
Precursor scoring | Relevance Heuristic |
---|---|
Min. plausibility | 0.01 |
Model | Template_relevance |
Template Set | Pistachio/Bkms_metabolic/Pistachio_ringbreaker/Reaxys/Reaxys_biocatalysis |
Top-N result to add to graph | 6 |
Feasible Synthetic Routes
Disclaimer and Information on In-Vitro Research Products
Please be aware that all articles and product information presented on BenchChem are intended solely for informational purposes. The products available for purchase on BenchChem are specifically designed for in-vitro studies, which are conducted outside of living organisms. In-vitro studies, derived from the Latin term "in glass," involve experiments performed in controlled laboratory settings using cells or tissues. It is important to note that these products are not categorized as medicines or drugs, and they have not received approval from the FDA for the prevention, treatment, or cure of any medical condition, ailment, or disease. We must emphasize that any form of bodily introduction of these products into humans or animals is strictly prohibited by law. It is essential to adhere to these guidelines to ensure compliance with legal and ethical standards in research and experimentation.