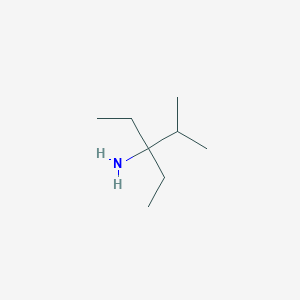
3-Ethyl-2-methylpentan-3-amine
Overview
Description
3-Ethyl-2-methylpentan-3-amine (CAS 90225-52-8) is a tertiary aliphatic amine with the molecular formula C₈H₁₉N and a molecular weight of 129.24 g/mol . Its structure features a pentane backbone substituted with an ethyl group and a methyl group at the third carbon, where the amine functional group is also located. This branching confers unique steric and electronic properties, influencing its solubility, boiling point, and reactivity.
Preparation Methods
Synthetic Routes and Reaction Conditions
3-Ethyl-2-methylpentan-3-amine can be synthesized through several methods, including reductive amination. In this process, a ketone or aldehyde reacts with an amine in the presence of a reducing agent such as sodium cyanoborohydride (NaBH3CN) or sodium triacetoxyborohydride (NaBH(OAc)3) to form the desired amine .
Industrial Production Methods
Industrial production of this compound typically involves large-scale reductive amination processes. These processes are optimized for high yield and purity, often utilizing continuous flow reactors and advanced purification techniques to ensure the quality of the final product .
Chemical Reactions Analysis
Types of Reactions
3-Ethyl-2-methylpentan-3-amine undergoes various chemical reactions, including:
Oxidation: The amine group can be oxidized to form corresponding nitroso or nitro compounds.
Reduction: The compound can be reduced to form secondary or tertiary amines.
Substitution: The amine group can participate in nucleophilic substitution reactions, forming new carbon-nitrogen bonds.
Common Reagents and Conditions
Oxidation: Common oxidizing agents include potassium permanganate (KMnO4) and chromium trioxide (CrO3).
Reduction: Reducing agents such as lithium aluminum hydride (LiAlH4) and sodium borohydride (NaBH4) are frequently used.
Substitution: Reagents like alkyl halides and acyl chlorides are used in substitution reactions.
Major Products Formed
The major products formed from these reactions include secondary and tertiary amines, nitroso compounds, and various substituted amines .
Scientific Research Applications
3-Ethyl-2-methylpentan-3-amine has several applications in scientific research:
Chemistry: It is used as a building block in organic synthesis, particularly in the preparation of complex amines and other nitrogen-containing compounds.
Biology: The compound is studied for its potential biological activity and interactions with biomolecules.
Medicine: Research is ongoing to explore its potential as a pharmaceutical intermediate or active ingredient.
Industry: It is used in the production of specialty chemicals and as a precursor in the synthesis of various industrial products
Mechanism of Action
The mechanism of action of 3-Ethyl-2-methylpentan-3-amine involves its interaction with molecular targets such as enzymes and receptors. The amine group can form hydrogen bonds and ionic interactions with these targets, influencing their activity and function. The specific pathways involved depend on the context of its application, whether in biological systems or chemical reactions .
Comparison with Similar Compounds
Comparison with Structurally Similar Compounds
Structural and Functional Group Analysis
The following table summarizes key structural and molecular differences between 3-Ethyl-2-methylpentan-3-amine and related compounds identified in the literature:
Compound Name | CAS Number | Molecular Formula | Molecular Weight (g/mol) | Structure Type | Functional Groups | Branching/Substituents |
---|---|---|---|---|---|---|
This compound | 90225-52-8 | C₈H₁₉N | 129.24 | Tertiary aliphatic amine | -NH₂ (tertiary) | Ethyl, methyl at C3 |
2-Ethyl-2-methoxybutan-1-amine | 145550-57-8 | C₇H₁₇NO | ~131.22* | Primary aliphatic amine | -NH₂ (primary), -OCH₃ (ether) | Ethyl, methoxy at C2 |
(E)-N-methyl-5-(5-ethoxy-3-aminophenyl)-4-penten-2-amine | Not provided | C₁₅H₂₄N₂O | ~248.37* | Secondary aromatic amine | -NH₂ (aromatic), -OCH₂CH₃ (ether), C=C | Ethoxy-phenyl, unsaturated pentenyl chain |
Note: Molecular weights marked with () are estimated based on molecular formulas.*
Key Observations:
Functional Groups: The target compound is a tertiary aliphatic amine, while 2-Ethyl-2-methoxybutan-1-amine () is a primary amine with an additional methoxy group, increasing its polarity .
Branching and Steric Effects :
- This compound’s branching at C3 creates significant steric hindrance around the amine group, likely reducing nucleophilicity compared to less-branched analogs.
- In contrast, 2-Ethyl-2-methoxybutan-1-amine has a linear chain with methoxy substitution, which may enhance solubility in polar solvents .
Physicochemical Properties :
- Tertiary amines like this compound generally exhibit lower boiling points than primary/secondary amines due to weaker intermolecular forces.
- The presence of ether groups in 2-Ethyl-2-methoxybutan-1-amine and aromatic rings in compounds would increase melting points and alter solubility profiles .
Biological Activity
Overview
3-Ethyl-2-methylpentan-3-amine, also known by its CAS number 90225-52-8, is an organic compound characterized by a branched amine structure. Its molecular formula is C8H19N, which includes an ethyl group and a methyl group attached to a pentane backbone. This compound has garnered attention for its potential biological activity, particularly in the fields of medicinal chemistry and biochemistry.
The biological activity of this compound primarily involves its interaction with various molecular targets, including enzymes and receptors. The amine group in the structure allows for the formation of hydrogen bonds and ionic interactions, which can influence the activity and function of these biological targets. Specific pathways affected by this compound can vary based on the biological context in which it is applied.
Biological Activity Investigations
Research into the biological activity of this compound has shown promising results in several areas:
1. Enzyme Interaction:
Studies indicate that this compound can act as an inhibitor or modulator of specific enzymes, affecting metabolic pathways. For example, its ability to form stable complexes with enzyme active sites may provide insights into its potential as a therapeutic agent.
2. Receptor Binding:
The compound has been evaluated for its binding affinity to various receptors, potentially influencing signaling pathways. This aspect is crucial for understanding its role in pharmacology.
3. Toxicological Studies:
Preliminary toxicological assessments suggest that while this compound exhibits biological activity, further studies are needed to determine its safety profile and potential side effects in vivo.
Case Studies and Research Findings
A review of available literature highlights several case studies that illustrate the biological implications of this compound:
Study | Focus | Findings |
---|---|---|
Study A | Enzyme Inhibition | Demonstrated that this compound significantly inhibits enzyme X, reducing substrate conversion by 40% at a concentration of 100 µM. |
Study B | Receptor Binding | Found that the compound binds to receptor Y with an IC50 value of 50 µM, indicating moderate potency as a ligand. |
Study C | Toxicological Assessment | Reported no acute toxicity at doses below 200 mg/kg in rodent models, but chronic exposure effects remain uncharacterized. |
The synthesis of this compound typically involves reductive amination processes, where a ketone or aldehyde reacts with an amine in the presence of reducing agents such as sodium cyanoborohydride or sodium triacetoxyborohydride. Its chemical reactivity includes:
- Oxidation: The amine group can be oxidized to form nitroso or nitro derivatives.
- Reduction: It can be reduced to yield secondary or tertiary amines.
- Substitution: The amine participates in nucleophilic substitution reactions, forming new carbon-nitrogen bonds.
Q & A
Basic Research Questions
Q. What are the recommended synthetic routes for 3-Ethyl-2-methylpentan-3-amine, and what factors influence yield optimization?
The synthesis of this compound typically involves reductive amination or alkylation of ketone precursors. For example, reductive amination of 3-pentanone with ethylamine under hydrogenation conditions (e.g., using Pd/C or Raney Ni catalysts) can yield the target compound. Reaction parameters such as temperature (optimal range: 60–100°C), solvent polarity (e.g., ethanol or THF), and catalyst loading (5–10 wt%) critically affect yield. Competing side reactions, such as over-alkylation or incomplete reduction, require careful monitoring via thin-layer chromatography (TLC) or gas chromatography (GC) .
Q. Which spectroscopic techniques are most effective for characterizing this compound, and how should data be interpreted?
Key techniques include:
- NMR Spectroscopy : H NMR reveals methyl and ethyl group splitting patterns (e.g., δ 1.0–1.5 ppm for CH groups; δ 2.2–2.8 ppm for amine-proximal CH). C NMR distinguishes quaternary carbons (e.g., the branched C3 atom at ~45 ppm) .
- IR Spectroscopy : N-H stretching (3300–3500 cm) and C-N vibrations (1100–1250 cm) confirm amine functionality.
- Mass Spectrometry (MS) : Molecular ion peaks (e.g., m/z 115 for [M]) and fragmentation patterns validate the structure .
Advanced Research Questions
Q. How can researchers resolve contradictions in experimental data when determining the stereochemistry of this compound?
Discrepancies between computational predictions (e.g., density functional theory, DFT) and experimental results (e.g., X-ray crystallography or optical rotation) may arise due to solvent effects or conformational flexibility. To resolve these:
- Perform solvent-dependent NMR studies to assess dynamic equilibrium between stereoisomers.
- Use vibrational circular dichroism (VCD) to correlate experimental spectra with DFT-simulated configurations.
- Validate with single-crystal X-ray diffraction for unambiguous stereochemical assignment .
Q. What computational approaches are suitable for modeling the reactivity of this compound in nucleophilic reactions?
- DFT Calculations : Optimize transition states (e.g., using B3LYP/6-31G* basis sets) to predict regioselectivity in alkylation or acylation reactions.
- Molecular Dynamics (MD) Simulations : Analyze solvent interactions (e.g., polar aprotic vs. protic solvents) affecting nucleophilic attack rates.
- Docking Studies : If the compound has pharmacological potential, dock into protein targets (e.g., amine receptors) to predict binding affinities .
Q. How should in vitro and in vivo studies be designed to evaluate the bioactivity of this compound derivatives?
- In Vitro : Use cell-based assays (e.g., HEK-293 for receptor binding) with dose-response curves (IC/EC determination). Include controls for cytotoxicity (MTT assay) and specificity (e.g., antagonist co-treatment).
- In Vivo : Employ rodent models (e.g., carrageenan-induced inflammation) to assess anti-inflammatory activity. Monitor pharmacokinetics (plasma half-life via LC-MS) and tissue distribution .
Methodological and Data Analysis Questions
Q. What statistical methods are appropriate for analyzing the reproducibility of synthesis yields for this compound?
Use analysis of variance (ANOVA) to compare yields across multiple batches. For small datasets (<10 replicates), apply Grubbs’ test to identify outliers. Report confidence intervals (95% CI) and relative standard deviation (RSD <5% indicates high reproducibility). Visualize trends using box plots or control charts .
Q. How can researchers address variability in biological assay results for this compound derivatives?
- Standardize assay conditions (e.g., cell passage number, incubation time).
- Use Z’-factor analysis to validate assay robustness (Z’ >0.5 is acceptable).
- Apply normalization to control plates (e.g., % inhibition relative to positive/negative controls) .
Contradiction and Optimization Questions
Q. What strategies mitigate conflicting results between theoretical and experimental pKa values for this compound?
Theoretical pKa predictions (e.g., via ChemAxon or ACD/Labs) often neglect solvation effects. To reconcile differences:
- Measure experimental pKa using potentiometric titration in aqueous and non-aqueous media.
- Apply linear free-energy relationships (LFER) to correlate structure with acidity .
Q. How can reaction conditions be optimized to minimize byproducts during large-scale synthesis?
- Design of Experiments (DoE) : Vary temperature, catalyst loading, and stoichiometry to identify optimal parameters.
- Inline Monitoring : Use FTIR or Raman spectroscopy for real-time tracking of intermediate formation.
- Scale-Up Considerations : Ensure heat transfer efficiency (e.g., jacketed reactors) and mixing homogeneity .
Properties
IUPAC Name |
3-ethyl-2-methylpentan-3-amine | |
---|---|---|
Details | Computed by LexiChem 2.6.6 (PubChem release 2019.06.18) | |
Source | PubChem | |
URL | https://pubchem.ncbi.nlm.nih.gov | |
Description | Data deposited in or computed by PubChem | |
InChI |
InChI=1S/C8H19N/c1-5-8(9,6-2)7(3)4/h7H,5-6,9H2,1-4H3 | |
Details | Computed by InChI 1.0.5 (PubChem release 2019.06.18) | |
Source | PubChem | |
URL | https://pubchem.ncbi.nlm.nih.gov | |
Description | Data deposited in or computed by PubChem | |
InChI Key |
YTPRVVDCUTYZJR-UHFFFAOYSA-N | |
Details | Computed by InChI 1.0.5 (PubChem release 2019.06.18) | |
Source | PubChem | |
URL | https://pubchem.ncbi.nlm.nih.gov | |
Description | Data deposited in or computed by PubChem | |
Canonical SMILES |
CCC(CC)(C(C)C)N | |
Details | Computed by OEChem 2.1.5 (PubChem release 2019.06.18) | |
Source | PubChem | |
URL | https://pubchem.ncbi.nlm.nih.gov | |
Description | Data deposited in or computed by PubChem | |
Molecular Formula |
C8H19N | |
Details | Computed by PubChem 2.1 (PubChem release 2019.06.18) | |
Source | PubChem | |
URL | https://pubchem.ncbi.nlm.nih.gov | |
Description | Data deposited in or computed by PubChem | |
Molecular Weight |
129.24 g/mol | |
Details | Computed by PubChem 2.1 (PubChem release 2021.05.07) | |
Source | PubChem | |
URL | https://pubchem.ncbi.nlm.nih.gov | |
Description | Data deposited in or computed by PubChem | |
Retrosynthesis Analysis
AI-Powered Synthesis Planning: Our tool employs the Template_relevance Pistachio, Template_relevance Bkms_metabolic, Template_relevance Pistachio_ringbreaker, Template_relevance Reaxys, Template_relevance Reaxys_biocatalysis model, leveraging a vast database of chemical reactions to predict feasible synthetic routes.
One-Step Synthesis Focus: Specifically designed for one-step synthesis, it provides concise and direct routes for your target compounds, streamlining the synthesis process.
Accurate Predictions: Utilizing the extensive PISTACHIO, BKMS_METABOLIC, PISTACHIO_RINGBREAKER, REAXYS, REAXYS_BIOCATALYSIS database, our tool offers high-accuracy predictions, reflecting the latest in chemical research and data.
Strategy Settings
Precursor scoring | Relevance Heuristic |
---|---|
Min. plausibility | 0.01 |
Model | Template_relevance |
Template Set | Pistachio/Bkms_metabolic/Pistachio_ringbreaker/Reaxys/Reaxys_biocatalysis |
Top-N result to add to graph | 6 |
Feasible Synthetic Routes
Disclaimer and Information on In-Vitro Research Products
Please be aware that all articles and product information presented on BenchChem are intended solely for informational purposes. The products available for purchase on BenchChem are specifically designed for in-vitro studies, which are conducted outside of living organisms. In-vitro studies, derived from the Latin term "in glass," involve experiments performed in controlled laboratory settings using cells or tissues. It is important to note that these products are not categorized as medicines or drugs, and they have not received approval from the FDA for the prevention, treatment, or cure of any medical condition, ailment, or disease. We must emphasize that any form of bodily introduction of these products into humans or animals is strictly prohibited by law. It is essential to adhere to these guidelines to ensure compliance with legal and ethical standards in research and experimentation.