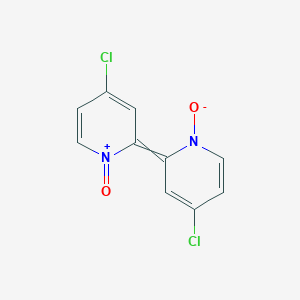
4,4'-Dichloro-2,2'-bipyridine N,N'-dioxide
- Click on QUICK INQUIRY to receive a quote from our team of experts.
- With the quality product at a COMPETITIVE price, you can focus more on your research.
Overview
Description
“4,4’-Dichloro-2,2’-bipyridine N,N’-dioxide” is a chemical compound with the molecular formula C10H6Cl2N2O2 . It is a derivative of 4,4’-Dichloro-2,2’-bipyridine, which has the molecular formula C10H6Cl2N2 .
Molecular Structure Analysis
The molecular structure of “4,4’-Dichloro-2,2’-bipyridine N,N’-dioxide” consists of 10 carbon atoms, 6 hydrogen atoms, 2 chlorine atoms, 2 nitrogen atoms, and 2 oxygen atoms . The average mass of the molecule is 225.074 Da .Scientific Research Applications
Catalysis in Carbon Dioxide Reduction
4,4'-Dichloro-2,2'-bipyridine N,N'-dioxide has been employed in catalytic studies related to carbon dioxide reduction. For example, a study by Smieja and Kubiak (2010) demonstrated that certain Re(bipy)(CO)3Cl complexes, including variants with 4,4'-dichloro-2,2'-bipyridine, exhibit improved catalytic activity for the reduction of carbon dioxide to carbon monoxide (Smieja & Kubiak, 2010).
Molecular Adduct Formation
The compound has also been investigated for its ability to form molecular adducts. A study by Arora, Talwelkar, and Pedireddi (2009) reported on the formation of molecular adducts of 4,4'-bipyridine N,N'-dioxide with various acids, demonstrating its potential in creating diverse molecular structures (Arora, Talwelkar, & Pedireddi, 2009).
Construction of Metal Coordination Networks
Long, Blake, Champness, and Schröder (2000) explored the use of 4,4'-bipyridine N,N'-dioxide in constructing metal coordination networks. Their research demonstrated its ability to engage in multiple binding modes, including M-L coordination bonds and hydrogen bonding, which is crucial in crystal engineering (Long et al., 2000).
Chemoenzymatic Synthesis
In the field of organic chemistry, this compound has been used in chemoenzymatic synthesis. Boyd et al. (2010) utilized it in the synthesis of chiral 2,2'-bipyridine ligands and their N,N'-dioxide derivatives for applications in asymmetric aminolysis of epoxides and asymmetric allylation of aldehydes (Boyd et al., 2010).
Catalysis in Asymmetric Reactions
The compound's derivatives have been used in catalysis for asymmetric chemical reactions. Bednářová et al. (2018) developed new axially chiral bipyridine N,N'-dioxides, employing them as catalysts in enantioselective allylation and aldol reactions, highlighting their significance in stereochemical control in organic synthesis (Bednářová et al., 2018).
Coordination Frameworks
The utility of 4,4'-bipyridine N,N'-dioxide in forming metal-ligand coordination frameworks was also researched by Long et al. (2000). They reported on coordination frameworks involving lanthanide elements, which demonstrate potential applications in materials science (Long et al., 2000).
Safety and Hazards
While specific safety and hazard information for “4,4’-Dichloro-2,2’-bipyridine N,N’-dioxide” is not available, it’s important to note that similar compounds can be harmful if swallowed, cause skin and eye irritation, and may cause respiratory irritation . Dust can form an explosive mixture with air, and thermal decomposition can lead to the release of irritating gases and vapors .
Properties
IUPAC Name |
4-chloro-2-(4-chloro-1-oxidopyridin-2-ylidene)pyridin-1-ium 1-oxide |
Source
|
---|---|---|
Details | Computed by LexiChem 2.6.6 (PubChem release 2019.06.18) | |
Source | PubChem | |
URL | https://pubchem.ncbi.nlm.nih.gov | |
Description | Data deposited in or computed by PubChem | |
InChI |
InChI=1S/C10H6Cl2N2O2/c11-7-1-3-13(15)9(5-7)10-6-8(12)2-4-14(10)16/h1-6H |
Source
|
Details | Computed by InChI 1.0.5 (PubChem release 2019.06.18) | |
Source | PubChem | |
URL | https://pubchem.ncbi.nlm.nih.gov | |
Description | Data deposited in or computed by PubChem | |
InChI Key |
TYRWLTCAPVOCMV-UHFFFAOYSA-N |
Source
|
Details | Computed by InChI 1.0.5 (PubChem release 2019.06.18) | |
Source | PubChem | |
URL | https://pubchem.ncbi.nlm.nih.gov | |
Description | Data deposited in or computed by PubChem | |
Canonical SMILES |
C1=CN(C(=C2C=C(C=C[N+]2=O)Cl)C=C1Cl)[O-] |
Source
|
Details | Computed by OEChem 2.1.5 (PubChem release 2019.06.18) | |
Source | PubChem | |
URL | https://pubchem.ncbi.nlm.nih.gov | |
Description | Data deposited in or computed by PubChem | |
Molecular Formula |
C10H6Cl2N2O2 |
Source
|
Details | Computed by PubChem 2.1 (PubChem release 2019.06.18) | |
Source | PubChem | |
URL | https://pubchem.ncbi.nlm.nih.gov | |
Description | Data deposited in or computed by PubChem | |
DSSTOX Substance ID |
DTXSID00722659 |
Source
|
Record name | 4-Chloro-2-(4-chloro-1-oxopyridin-1-ium-2(1H)-ylidene)pyridin-1(2H)-olate | |
Source | EPA DSSTox | |
URL | https://comptox.epa.gov/dashboard/DTXSID00722659 | |
Description | DSSTox provides a high quality public chemistry resource for supporting improved predictive toxicology. | |
Molecular Weight |
257.07 g/mol |
Source
|
Details | Computed by PubChem 2.1 (PubChem release 2021.05.07) | |
Source | PubChem | |
URL | https://pubchem.ncbi.nlm.nih.gov | |
Description | Data deposited in or computed by PubChem | |
CAS No. |
84175-08-6 |
Source
|
Record name | 4-Chloro-2-(4-chloro-1-oxopyridin-1-ium-2(1H)-ylidene)pyridin-1(2H)-olate | |
Source | EPA DSSTox | |
URL | https://comptox.epa.gov/dashboard/DTXSID00722659 | |
Description | DSSTox provides a high quality public chemistry resource for supporting improved predictive toxicology. | |
Disclaimer and Information on In-Vitro Research Products
Please be aware that all articles and product information presented on BenchChem are intended solely for informational purposes. The products available for purchase on BenchChem are specifically designed for in-vitro studies, which are conducted outside of living organisms. In-vitro studies, derived from the Latin term "in glass," involve experiments performed in controlled laboratory settings using cells or tissues. It is important to note that these products are not categorized as medicines or drugs, and they have not received approval from the FDA for the prevention, treatment, or cure of any medical condition, ailment, or disease. We must emphasize that any form of bodily introduction of these products into humans or animals is strictly prohibited by law. It is essential to adhere to these guidelines to ensure compliance with legal and ethical standards in research and experimentation.