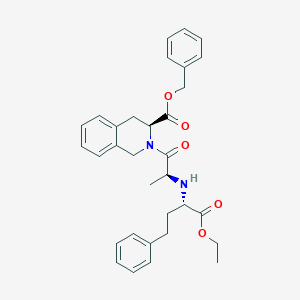
Quinapril benzyl ester
Overview
Description
Quinapril benzyl ester is a chemical compound with the molecular formula C32H36N2O5 . It is a white to off-white crystalline solid with good solubility properties. This compound is a prodrug of quinapril, an angiotensin-converting enzyme (ACE) inhibitor used in the treatment of hypertension and heart failure. Upon administration, it is hydrolyzed in the body to release the active drug, quinapril .
Mechanism of Action
Biochemical Pathways
The primary biochemical pathway affected by quinapril benzyl ester is the RAAS. By inhibiting ACE, quinaprilat disrupts this system, leading to a decrease in angiotensin II levels . This results in vasodilation and a reduction in the volume of circulating fluid, which lowers blood pressure .
Pharmacokinetics
Quinapril is rapidly absorbed and de-esterified to quinaprilat, the active metabolite . The pharmacokinetics of quinapril and quinaprilat can be affected by renal and hepatic impairment . In patients with hypertension or congestive heart failure, no increase in adverse events was observed when the dosage was increased from 10 to 40 mg/day .
Action Environment
The action of quinapril can be influenced by various environmental factors. For instance, the presence of food in the gastrointestinal tract can affect the absorption of quinapril . Additionally, the drug’s efficacy and stability can be affected by factors such as temperature and pH.
Biochemical Analysis
Biochemical Properties
Quinapril Benzyl Ester plays a significant role in biochemical reactions, particularly in the renin-angiotensin system . It interacts with various enzymes and proteins, most notably the angiotensin-converting enzyme (ACE). The compound is rapidly de-esterified after absorption to form Quinaprilat, the active diacid metabolite . This interaction is crucial in mediating the compound’s effects on blood pressure regulation and cardiovascular function .
Cellular Effects
This compound exerts several effects on cellular processes. It influences cell function by impacting cell signaling pathways, gene expression, and cellular metabolism . As an ACE inhibitor, it reduces the production of angiotensin II, a potent vasoconstrictor, thereby promoting vasodilation and reducing blood pressure . It also inhibits the degradation of bradykinin, a vasodilator, further contributing to its antihypertensive effects .
Molecular Mechanism
The molecular mechanism of action of this compound involves binding interactions with biomolecules, enzyme inhibition, and changes in gene expression . Upon absorption, it is converted to Quinaprilat, which binds to and inhibits ACE . This prevents the conversion of angiotensin I to angiotensin II, reducing vasoconstriction and aldosterone secretion . The result is lowered blood pressure and decreased workload on the heart .
Temporal Effects in Laboratory Settings
In laboratory settings, the effects of this compound change over time. It is stable under normal conditions . The compound’s effects on cellular function, such as its antihypertensive action, may vary over time due to factors like drug metabolism and elimination .
Dosage Effects in Animal Models
In animal models, the effects of this compound vary with dosage . Lower doses effectively reduce blood pressure, while higher doses may lead to more pronounced effects . Excessively high doses could potentially lead to adverse effects, such as hypotension .
Metabolic Pathways
This compound is involved in the renin-angiotensin system metabolic pathway . It interacts with the enzyme ACE and undergoes de-esterification to form the active metabolite, Quinaprilat . This process is crucial for the compound’s antihypertensive effects .
Transport and Distribution
This compound is absorbed and distributed within cells and tissues after administration
Preparation Methods
Synthetic Routes and Reaction Conditions
The synthesis of quinapril benzyl ester typically involves the esterification of quinapril with benzyl alcohol. One common method includes the reaction of quinapril with benzyl alcohol in the presence of a strong acid catalyst, such as sulfuric acid, under reflux conditions. The reaction mixture is then neutralized, and the product is purified by recrystallization .
Industrial Production Methods
Industrial production of this compound follows similar synthetic routes but on a larger scale. The process involves the use of large reactors and continuous flow systems to ensure consistent product quality. The reaction conditions are optimized to maximize yield and minimize impurities. The final product is subjected to rigorous quality control measures to ensure it meets pharmaceutical standards .
Chemical Reactions Analysis
Types of Reactions
Quinapril benzyl ester undergoes several types of chemical reactions, including:
Hydrolysis: In the presence of water or aqueous acids, this compound is hydrolyzed to quinapril and benzyl alcohol.
Oxidation: this compound can be oxidized to form this compound oxide.
Reduction: Reduction reactions can convert this compound to its corresponding alcohol derivatives.
Common Reagents and Conditions
Hydrolysis: Typically carried out using dilute hydrochloric acid or sodium hydroxide at room temperature.
Oxidation: Common oxidizing agents include potassium permanganate and hydrogen peroxide.
Reduction: Reducing agents such as lithium aluminum hydride or sodium borohydride are used under controlled conditions.
Major Products Formed
Hydrolysis: Quinapril and benzyl alcohol.
Oxidation: this compound oxide.
Reduction: Alcohol derivatives of this compound.
Scientific Research Applications
Pharmaceutical Synthesis
Quinapril benzyl ester serves as an intermediate in the synthesis of quinapril hydrochloride, a more active form used in clinical settings. The synthesis process typically involves catalytic hydrogenolysis, where the benzyl ester is converted to quinapril hydrochloride under specific conditions. This method has been shown to yield high purity and good yield of the final product.
Synthesis Process Overview
- Starting Material : Benzyl ester of quinapril hydrochloride
- Catalyst : Palladium on carbon (Pd/C)
- Conditions :
- Pressure: 40 to 150 psi
- Temperature: 10-40 °C
- Yield : Approximately 85.5% with purity around 99.7% .
Analytical Method Development
This compound is utilized in the development of analytical methods for quality control (QC) and validation processes in pharmaceutical manufacturing. Its chemical properties make it suitable for use as a reference standard in various analytical techniques, including chromatography and mass spectrometry.
Key Applications in Analytical Testing
- Method Validation : Ensures that analytical methods produce reliable results.
- Quality Control : Monitors the consistency and quality of pharmaceutical products.
- Reference Standard : Acts as a benchmark for comparison during testing .
Case Studies
Several studies have documented the effectiveness of this compound in various applications:
Case Study 1: Synthesis Efficiency
A study demonstrated that using this compound in the synthesis of quinapril hydrochloride reduced impurities significantly, with diketopiperazine levels dropping below 0.2%. This efficiency is crucial for producing high-quality pharmaceuticals .
Case Study 2: Impurity Profiling
Research highlighted the role of this compound in identifying process-related impurities during drug formulation. This profiling is essential for ensuring patient safety and drug efficacy .
Comparison with Similar Compounds
Similar Compounds
Quinapril hydrochloride: Another ester form of quinapril, used for similar therapeutic purposes.
Quinapril ethyl ester: An ester derivative of quinapril with similar pharmacological properties.
Quinapril methyl ester: Another ester form with comparable effects.
Uniqueness
Quinapril benzyl ester is unique due to its specific ester linkage, which influences its hydrolysis rate and bioavailability. Compared to other ester derivatives, this compound may offer different pharmacokinetic properties, making it a valuable compound for specific therapeutic applications .
Biological Activity
Quinapril benzyl ester is a derivative of quinapril, an angiotensin-converting enzyme (ACE) inhibitor used primarily in the treatment of hypertension and heart failure. This article explores the biological activity of this compound, focusing on its pharmacological effects, mechanisms of action, and relevant research findings.
Overview of this compound
This compound is synthesized from quinapril and is characterized by its ability to inhibit ACE, leading to decreased production of angiotensin II. This results in vasodilation and reduced blood pressure. The compound's structure can be represented as follows:
- Chemical Formula : C₃₂H₃₆N₂O₅
- Molecular Weight : 520.65 g/mol
Quinapril acts as a prodrug, which is converted to its active form, quinaprilat, after administration. The mechanism involves the inhibition of ACE, an enzyme responsible for converting angiotensin I to angiotensin II. The inhibition leads to several biological effects:
- Decreased Blood Pressure : By reducing angiotensin II levels, quinapril decreases vascular resistance.
- Decreased Aldosterone Production : This results in reduced sodium retention and fluid volume.
- Enhanced Bradykinin Levels : Inhibition of ACE also prevents the breakdown of bradykinin, a vasodilator.
Pharmacokinetics
The pharmacokinetic profile of this compound includes:
- Bioavailability : 50-80% after oral administration.
- Peak Plasma Concentration (Tmax) : Less than 1 hour for quinapril; approximately 2.5 hours for quinaprilat.
- Half-life : Approximately 2.3 hours for quinaprilat.
- Protein Binding : About 97% bound to plasma proteins.
Antihypertensive Effects
Research indicates that this compound effectively lowers blood pressure in hypertensive models. A study demonstrated that doses ranging from 10 mg to 80 mg daily significantly reduced systolic and diastolic blood pressure in patients with hypertension .
Cardiovascular Benefits
In addition to its antihypertensive effects, quinapril has been shown to reduce the incidence of ischemic events in patients undergoing coronary artery bypass grafting (CABG) . The compound's ability to improve endothelial function and reduce myocardial oxygen demand contributes to its cardioprotective properties.
Case Studies and Research Findings
- Case Study on Hypertension Management :
- Meta-analysis on ACE Inhibitors :
- In Vitro Studies on Enzyme Inhibition :
Comparative Analysis Table
Property | This compound | Quinapril |
---|---|---|
Chemical Structure | C₃₂H₃₆N₂O₅ | C₂₁H₂₄N₂O₅ |
Bioavailability | 50-80% | 60-80% |
Peak Plasma Concentration | <1 hour | <1 hour |
Half-life | 2.3 hours | 2 hours |
Primary Use | Antihypertensive | Antihypertensive |
Properties
IUPAC Name |
benzyl (3S)-2-[(2S)-2-[[(2S)-1-ethoxy-1-oxo-4-phenylbutan-2-yl]amino]propanoyl]-3,4-dihydro-1H-isoquinoline-3-carboxylate | |
---|---|---|
Details | Computed by LexiChem 2.6.6 (PubChem release 2019.06.18) | |
Source | PubChem | |
URL | https://pubchem.ncbi.nlm.nih.gov | |
Description | Data deposited in or computed by PubChem | |
InChI |
InChI=1S/C32H36N2O5/c1-3-38-31(36)28(19-18-24-12-6-4-7-13-24)33-23(2)30(35)34-21-27-17-11-10-16-26(27)20-29(34)32(37)39-22-25-14-8-5-9-15-25/h4-17,23,28-29,33H,3,18-22H2,1-2H3/t23-,28-,29-/m0/s1 | |
Details | Computed by InChI 1.0.5 (PubChem release 2019.06.18) | |
Source | PubChem | |
URL | https://pubchem.ncbi.nlm.nih.gov | |
Description | Data deposited in or computed by PubChem | |
InChI Key |
XSOUEGBLZLHHGC-OIFPXGRLSA-N | |
Details | Computed by InChI 1.0.5 (PubChem release 2019.06.18) | |
Source | PubChem | |
URL | https://pubchem.ncbi.nlm.nih.gov | |
Description | Data deposited in or computed by PubChem | |
Canonical SMILES |
CCOC(=O)C(CCC1=CC=CC=C1)NC(C)C(=O)N2CC3=CC=CC=C3CC2C(=O)OCC4=CC=CC=C4 | |
Details | Computed by OEChem 2.1.5 (PubChem release 2019.06.18) | |
Source | PubChem | |
URL | https://pubchem.ncbi.nlm.nih.gov | |
Description | Data deposited in or computed by PubChem | |
Isomeric SMILES |
CCOC(=O)[C@H](CCC1=CC=CC=C1)N[C@@H](C)C(=O)N2CC3=CC=CC=C3C[C@H]2C(=O)OCC4=CC=CC=C4 | |
Details | Computed by OEChem 2.1.5 (PubChem release 2019.06.18) | |
Source | PubChem | |
URL | https://pubchem.ncbi.nlm.nih.gov | |
Description | Data deposited in or computed by PubChem | |
Molecular Formula |
C32H36N2O5 | |
Details | Computed by PubChem 2.1 (PubChem release 2019.06.18) | |
Source | PubChem | |
URL | https://pubchem.ncbi.nlm.nih.gov | |
Description | Data deposited in or computed by PubChem | |
Molecular Weight |
528.6 g/mol | |
Details | Computed by PubChem 2.1 (PubChem release 2021.05.07) | |
Source | PubChem | |
URL | https://pubchem.ncbi.nlm.nih.gov | |
Description | Data deposited in or computed by PubChem | |
Retrosynthesis Analysis
AI-Powered Synthesis Planning: Our tool employs the Template_relevance Pistachio, Template_relevance Bkms_metabolic, Template_relevance Pistachio_ringbreaker, Template_relevance Reaxys, Template_relevance Reaxys_biocatalysis model, leveraging a vast database of chemical reactions to predict feasible synthetic routes.
One-Step Synthesis Focus: Specifically designed for one-step synthesis, it provides concise and direct routes for your target compounds, streamlining the synthesis process.
Accurate Predictions: Utilizing the extensive PISTACHIO, BKMS_METABOLIC, PISTACHIO_RINGBREAKER, REAXYS, REAXYS_BIOCATALYSIS database, our tool offers high-accuracy predictions, reflecting the latest in chemical research and data.
Strategy Settings
Precursor scoring | Relevance Heuristic |
---|---|
Min. plausibility | 0.01 |
Model | Template_relevance |
Template Set | Pistachio/Bkms_metabolic/Pistachio_ringbreaker/Reaxys/Reaxys_biocatalysis |
Top-N result to add to graph | 6 |
Feasible Synthetic Routes
Disclaimer and Information on In-Vitro Research Products
Please be aware that all articles and product information presented on BenchChem are intended solely for informational purposes. The products available for purchase on BenchChem are specifically designed for in-vitro studies, which are conducted outside of living organisms. In-vitro studies, derived from the Latin term "in glass," involve experiments performed in controlled laboratory settings using cells or tissues. It is important to note that these products are not categorized as medicines or drugs, and they have not received approval from the FDA for the prevention, treatment, or cure of any medical condition, ailment, or disease. We must emphasize that any form of bodily introduction of these products into humans or animals is strictly prohibited by law. It is essential to adhere to these guidelines to ensure compliance with legal and ethical standards in research and experimentation.