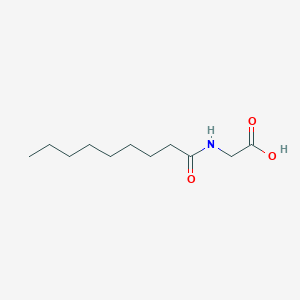
N-Nonanoylglycine
Overview
Description
N-Nonanoylglycine is a compound classified as an acylglycine, where the acyl group is derived from nonanoic acid. It is a minor metabolite of fatty acids and is typically found in the urine and blood of patients with various fatty acid oxidation disorders . The molecular formula of this compound is C11H21NO3, and it is known for its role as a metabolite in biological systems .
Preparation Methods
Synthetic Routes and Reaction Conditions: N-Nonanoylglycine can be synthesized through the reaction of nonanoic acid with glycine. The reaction typically involves the activation of nonanoic acid using a coupling reagent such as dicyclohexylcarbodiimide (DCC) in the presence of a catalyst like 4-dimethylaminopyridine (DMAP). The reaction is carried out in an organic solvent such as dichloromethane at room temperature. The product is then purified using standard techniques like recrystallization or chromatography .
Industrial Production Methods: Industrial production of this compound follows similar synthetic routes but on a larger scale. The process involves the use of automated reactors and continuous flow systems to ensure consistent quality and yield. The reaction conditions are optimized to maximize the efficiency and minimize the production costs .
Chemical Reactions Analysis
Types of Reactions: N-Nonanoylglycine undergoes various chemical reactions, including:
Oxidation: It can be oxidized to form corresponding carboxylic acids.
Reduction: Reduction reactions can convert it into primary amines.
Substitution: It can participate in nucleophilic substitution reactions, where the acyl group can be replaced by other functional groups
Common Reagents and Conditions:
Oxidation: Common oxidizing agents include potassium permanganate (KMnO4) and chromium trioxide (CrO3).
Reduction: Reducing agents such as lithium aluminum hydride (LiAlH4) and sodium borohydride (NaBH4) are used.
Substitution: Nucleophiles like ammonia (NH3) and primary amines are commonly used in substitution reactions
Major Products:
Oxidation: Nonanoic acid derivatives.
Reduction: Primary amines.
Substitution: Various substituted glycine derivatives
Scientific Research Applications
N-Nonanoylglycine has several applications in scientific research:
Chemistry: It is used as a model compound to study acylglycine metabolism and its role in fatty acid oxidation disorders.
Biology: It serves as a biomarker for diagnosing and monitoring metabolic disorders.
Medicine: Research on this compound contributes to understanding the pathophysiology of fatty acid oxidation disorders and developing therapeutic interventions.
Industry: It is used in the synthesis of specialty chemicals and pharmaceuticals .
Mechanism of Action
N-Nonanoylglycine exerts its effects primarily through its role as a metabolite in fatty acid oxidation. It is involved in the detoxification of fatty acids by conjugating with glycine, forming acylglycines that are excreted in the urine. This process helps in maintaining the balance of fatty acids in the body and preventing the accumulation of toxic intermediates .
Comparison with Similar Compounds
N-Decanoylglycine: Similar structure with a decanoyl group instead of a nonanoyl group.
N-Octanoylglycine: Similar structure with an octanoyl group instead of a nonanoyl group.
N-Heptanoylglycine: Similar structure with a heptanoyl group instead of a nonanoyl group
Uniqueness: N-Nonanoylglycine is unique due to its specific acyl group derived from nonanoic acid, which influences its metabolic pathways and biological roles. Its presence in biological systems is indicative of specific metabolic conditions, making it a valuable biomarker for certain disorders .
Properties
IUPAC Name |
2-(nonanoylamino)acetic acid | |
---|---|---|
Details | Computed by LexiChem 2.6.6 (PubChem release 2019.06.18) | |
Source | PubChem | |
URL | https://pubchem.ncbi.nlm.nih.gov | |
Description | Data deposited in or computed by PubChem | |
InChI |
InChI=1S/C11H21NO3/c1-2-3-4-5-6-7-8-10(13)12-9-11(14)15/h2-9H2,1H3,(H,12,13)(H,14,15) | |
Details | Computed by InChI 1.0.5 (PubChem release 2019.06.18) | |
Source | PubChem | |
URL | https://pubchem.ncbi.nlm.nih.gov | |
Description | Data deposited in or computed by PubChem | |
InChI Key |
JXDFEUKNHBHUCP-UHFFFAOYSA-N | |
Details | Computed by InChI 1.0.5 (PubChem release 2019.06.18) | |
Source | PubChem | |
URL | https://pubchem.ncbi.nlm.nih.gov | |
Description | Data deposited in or computed by PubChem | |
Canonical SMILES |
CCCCCCCCC(=O)NCC(=O)O | |
Details | Computed by OEChem 2.1.5 (PubChem release 2019.06.18) | |
Source | PubChem | |
URL | https://pubchem.ncbi.nlm.nih.gov | |
Description | Data deposited in or computed by PubChem | |
Molecular Formula |
C11H21NO3 | |
Details | Computed by PubChem 2.1 (PubChem release 2019.06.18) | |
Source | PubChem | |
URL | https://pubchem.ncbi.nlm.nih.gov | |
Description | Data deposited in or computed by PubChem | |
Molecular Weight |
215.29 g/mol | |
Details | Computed by PubChem 2.1 (PubChem release 2021.05.07) | |
Source | PubChem | |
URL | https://pubchem.ncbi.nlm.nih.gov | |
Description | Data deposited in or computed by PubChem | |
Physical Description |
Solid | |
Record name | N-Nonanoylglycine | |
Source | Human Metabolome Database (HMDB) | |
URL | http://www.hmdb.ca/metabolites/HMDB0013279 | |
Description | The Human Metabolome Database (HMDB) is a freely available electronic database containing detailed information about small molecule metabolites found in the human body. | |
Explanation | HMDB is offered to the public as a freely available resource. Use and re-distribution of the data, in whole or in part, for commercial purposes requires explicit permission of the authors and explicit acknowledgment of the source material (HMDB) and the original publication (see the HMDB citing page). We ask that users who download significant portions of the database cite the HMDB paper in any resulting publications. | |
Synthesis routes and methods I
Procedure details
Synthesis routes and methods II
Procedure details
Retrosynthesis Analysis
AI-Powered Synthesis Planning: Our tool employs the Template_relevance Pistachio, Template_relevance Bkms_metabolic, Template_relevance Pistachio_ringbreaker, Template_relevance Reaxys, Template_relevance Reaxys_biocatalysis model, leveraging a vast database of chemical reactions to predict feasible synthetic routes.
One-Step Synthesis Focus: Specifically designed for one-step synthesis, it provides concise and direct routes for your target compounds, streamlining the synthesis process.
Accurate Predictions: Utilizing the extensive PISTACHIO, BKMS_METABOLIC, PISTACHIO_RINGBREAKER, REAXYS, REAXYS_BIOCATALYSIS database, our tool offers high-accuracy predictions, reflecting the latest in chemical research and data.
Strategy Settings
Precursor scoring | Relevance Heuristic |
---|---|
Min. plausibility | 0.01 |
Model | Template_relevance |
Template Set | Pistachio/Bkms_metabolic/Pistachio_ringbreaker/Reaxys/Reaxys_biocatalysis |
Top-N result to add to graph | 6 |
Feasible Synthetic Routes
Disclaimer and Information on In-Vitro Research Products
Please be aware that all articles and product information presented on BenchChem are intended solely for informational purposes. The products available for purchase on BenchChem are specifically designed for in-vitro studies, which are conducted outside of living organisms. In-vitro studies, derived from the Latin term "in glass," involve experiments performed in controlled laboratory settings using cells or tissues. It is important to note that these products are not categorized as medicines or drugs, and they have not received approval from the FDA for the prevention, treatment, or cure of any medical condition, ailment, or disease. We must emphasize that any form of bodily introduction of these products into humans or animals is strictly prohibited by law. It is essential to adhere to these guidelines to ensure compliance with legal and ethical standards in research and experimentation.