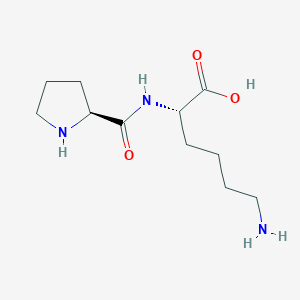
Pro-Lys
Overview
Description
Proline-lysine, commonly referred to as Pro-Lys, is a dipeptide composed of the amino acids proline and lysine. This compound is of significant interest in various fields due to its unique structural properties and biological activities. Proline is a cyclic amino acid, while lysine is a basic amino acid with an ε-amino group, making this compound a versatile molecule in biochemical and industrial applications.
Preparation Methods
Synthetic Routes and Reaction Conditions
Proline-lysine can be synthesized through standard peptide synthesis techniques. One common method involves the use of solid-phase peptide synthesis (SPPS), where the amino acids are sequentially added to a growing peptide chain anchored to a solid resin. The process typically involves the following steps:
Activation: The carboxyl group of proline is activated using a coupling reagent such as dicyclohexylcarbodiimide (DCC) or 1-ethyl-3-(3-dimethylaminopropyl)carbodiimide (EDC).
Coupling: The activated proline is then coupled to the amino group of lysine, forming the peptide bond.
Deprotection: Protective groups on the amino acids are removed to yield the final dipeptide.
Industrial Production Methods
In industrial settings, the production of Pro-Lys may involve enzymatic synthesis using proteases or peptidases that catalyze the formation of peptide bonds. This method is advantageous due to its specificity and mild reaction conditions, which help in maintaining the integrity of the amino acids.
Chemical Reactions Analysis
Types of Reactions
Proline-lysine undergoes various chemical reactions, including:
Oxidation: The ε-amino group of lysine can be oxidized to form aldehydes or carboxylic acids.
Reduction: Reduction reactions can convert the carbonyl groups in proline to hydroxyl groups.
Substitution: The amino groups in lysine can participate in nucleophilic substitution reactions.
Common Reagents and Conditions
Oxidation: Reagents such as hydrogen peroxide or potassium permanganate are commonly used.
Reduction: Sodium borohydride or lithium aluminum hydride are typical reducing agents.
Substitution: Alkyl halides or acyl chlorides are often used in substitution reactions.
Major Products
The major products of these reactions include hydroxylated derivatives, substituted amines, and various oxidized forms of the dipeptide.
Scientific Research Applications
Proline-lysine has a wide range of applications in scientific research:
Chemistry: Used as a building block in the synthesis of more complex peptides and proteins.
Biology: Plays a role in studying protein-protein interactions and enzyme-substrate specificity.
Medicine: Investigated for its potential in drug delivery systems and as a therapeutic agent in wound healing and tissue regeneration.
Industry: Utilized in the production of bioactive peptides for cosmetics and nutraceuticals.
Mechanism of Action
The mechanism of action of Pro-Lys involves its interaction with various molecular targets. The ε-amino group of lysine can form hydrogen bonds and ionic interactions with negatively charged residues in proteins, influencing their structure and function. Proline, with its cyclic structure, induces conformational constraints in peptides, affecting their stability and biological activity.
Comparison with Similar Compounds
Similar Compounds
Proline-arginine: Another dipeptide with similar structural properties but different biological activities due to the presence of arginine.
Proline-histidine: Contains an imidazole ring, offering different chemical reactivity and applications.
Proline-glycine: A simpler dipeptide with distinct flexibility and less steric hindrance.
Uniqueness
Proline-lysine is unique due to the combination of proline’s conformational rigidity and lysine’s basicity, making it a valuable compound in various biochemical and industrial applications.
Properties
IUPAC Name |
(2S)-6-amino-2-[[(2S)-pyrrolidine-2-carbonyl]amino]hexanoic acid | |
---|---|---|
Details | Computed by LexiChem 2.6.6 (PubChem release 2019.06.18) | |
Source | PubChem | |
URL | https://pubchem.ncbi.nlm.nih.gov | |
Description | Data deposited in or computed by PubChem | |
InChI |
InChI=1S/C11H21N3O3/c12-6-2-1-4-9(11(16)17)14-10(15)8-5-3-7-13-8/h8-9,13H,1-7,12H2,(H,14,15)(H,16,17)/t8-,9-/m0/s1 | |
Details | Computed by InChI 1.0.5 (PubChem release 2019.06.18) | |
Source | PubChem | |
URL | https://pubchem.ncbi.nlm.nih.gov | |
Description | Data deposited in or computed by PubChem | |
InChI Key |
RVQDZELMXZRSSI-IUCAKERBSA-N | |
Details | Computed by InChI 1.0.5 (PubChem release 2019.06.18) | |
Source | PubChem | |
URL | https://pubchem.ncbi.nlm.nih.gov | |
Description | Data deposited in or computed by PubChem | |
Canonical SMILES |
C1CC(NC1)C(=O)NC(CCCCN)C(=O)O | |
Details | Computed by OEChem 2.1.5 (PubChem release 2019.06.18) | |
Source | PubChem | |
URL | https://pubchem.ncbi.nlm.nih.gov | |
Description | Data deposited in or computed by PubChem | |
Isomeric SMILES |
C1C[C@H](NC1)C(=O)N[C@@H](CCCCN)C(=O)O | |
Details | Computed by OEChem 2.1.5 (PubChem release 2019.06.18) | |
Source | PubChem | |
URL | https://pubchem.ncbi.nlm.nih.gov | |
Description | Data deposited in or computed by PubChem | |
Molecular Formula |
C11H21N3O3 | |
Details | Computed by PubChem 2.1 (PubChem release 2019.06.18) | |
Source | PubChem | |
URL | https://pubchem.ncbi.nlm.nih.gov | |
Description | Data deposited in or computed by PubChem | |
Molecular Weight |
243.30 g/mol | |
Details | Computed by PubChem 2.1 (PubChem release 2021.05.07) | |
Source | PubChem | |
URL | https://pubchem.ncbi.nlm.nih.gov | |
Description | Data deposited in or computed by PubChem | |
Retrosynthesis Analysis
AI-Powered Synthesis Planning: Our tool employs the Template_relevance Pistachio, Template_relevance Bkms_metabolic, Template_relevance Pistachio_ringbreaker, Template_relevance Reaxys, Template_relevance Reaxys_biocatalysis model, leveraging a vast database of chemical reactions to predict feasible synthetic routes.
One-Step Synthesis Focus: Specifically designed for one-step synthesis, it provides concise and direct routes for your target compounds, streamlining the synthesis process.
Accurate Predictions: Utilizing the extensive PISTACHIO, BKMS_METABOLIC, PISTACHIO_RINGBREAKER, REAXYS, REAXYS_BIOCATALYSIS database, our tool offers high-accuracy predictions, reflecting the latest in chemical research and data.
Strategy Settings
Precursor scoring | Relevance Heuristic |
---|---|
Min. plausibility | 0.01 |
Model | Template_relevance |
Template Set | Pistachio/Bkms_metabolic/Pistachio_ringbreaker/Reaxys/Reaxys_biocatalysis |
Top-N result to add to graph | 6 |
Feasible Synthetic Routes
Disclaimer and Information on In-Vitro Research Products
Please be aware that all articles and product information presented on BenchChem are intended solely for informational purposes. The products available for purchase on BenchChem are specifically designed for in-vitro studies, which are conducted outside of living organisms. In-vitro studies, derived from the Latin term "in glass," involve experiments performed in controlled laboratory settings using cells or tissues. It is important to note that these products are not categorized as medicines or drugs, and they have not received approval from the FDA for the prevention, treatment, or cure of any medical condition, ailment, or disease. We must emphasize that any form of bodily introduction of these products into humans or animals is strictly prohibited by law. It is essential to adhere to these guidelines to ensure compliance with legal and ethical standards in research and experimentation.