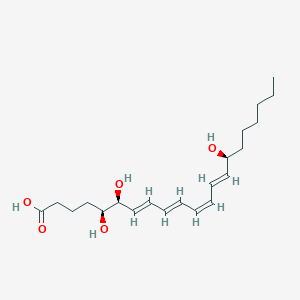
lipoxin A4
Overview
Description
Lipoxin A4 (5S,6R,15S-trihydroxy-7E,9E,11Z,13E-eicosatetraenoic acid) is a bioactive lipid mediator derived from arachidonic acid. It is known for its potent anti-inflammatory and immunomodulatory properties. This compound plays a crucial role in the resolution of inflammation by promoting the clearance of apoptotic neutrophils and regulating the behavior of various immune cells, including neutrophils, macrophages, and T and B cells .
Mechanism of Action
Lipoxin A4 (LXA4) is an endogenous bioactive lipid mediator involved in the regulation of inflammation . This article will explore the mechanism of action of LXA4, including its targets, mode of action, biochemical pathways, pharmacokinetics, results of action, and the influence of environmental factors on its action.
Target of Action
LXA4 exerts immunomodulatory effects by regulating the behavior of various immune cells, including neutrophils, macrophages, and T and B cells . It promotes the clearance of apoptotic neutrophils, helping to dampen inflammation and promote tissue repair .
Mode of Action
LXA4 interacts with its targets to regulate the expression of many inflammatory genes. It modulates the levels of transcription factors, such as nuclear factor κB (NF-κB), activator protein-1 (AP-1), nerve growth factor-regulated factor 1A binding protein 1 (NGF), and peroxisome proliferator activated receptor γ (PPAR-γ) . These transcription factors are elevated in various diseases, such as respiratory tract diseases, renal diseases, cancer, neurodegenerative diseases, and viral infections .
Biochemical Pathways
LXA4 is involved in the regulation of several biochemical pathways. For instance, in cases of obesity-related glomerulopathy, the administration of LXA4 markedly reduces inflammatory stimuli by inhibiting the Nfkbia and Mapk1/14 pathways, leading to the downregulation of Il1b, Il6, Tnf, and Ccl8 . This indicates an anti-inflammatory response .
Pharmacokinetics
It is known that lxa4 is produced enzymatically through the sequential metabolism of the lipoxygenase (lo) enzymes 5-lo and either 12-lo or 15-lo . Aspirin directly affects the LXA4 circuit by activating the biosynthesis of endogenous epimers of LXA4, known as aspirin-triggered 15-epi-LX, which has potent anti-inflammatory properties .
Result of Action
The administration of LXA4 results in significant anti-inflammatory and neuroprotective effects. For instance, in a rat model of middle cerebral artery occlusion (MCAO) and BV2 microglial cells, LXA4 inhibited the pro-inflammatory response and exerted a significant neuroprotective effect through the downregulation of Tnf, Il1b, Fcgr2a, Notch1, Hes1, Nos2 and activation of Arg1, Mrc1, Hes5 genes .
Biochemical Analysis
Biochemical Properties
Lipoxin A4 exerts immunomodulatory effects by regulating the behavior of various immune cells, including neutrophils, macrophages, and T and B cells . It promotes the clearance of apoptotic neutrophils, helping to dampen inflammation and promote tissue repair . This compound regulates the expression of many inflammatory genes by modulating the levels of transcription factors, such as nuclear factor κB (NF-κB), activator protein-1 (AP-1), nerve growth factor-regulated factor 1A binding protein 1 (NGF), and peroxisome proliferator activated receptor γ (PPAR-γ) .
Cellular Effects
This compound influences cell function by affecting cell signaling pathways, gene expression, and cellular metabolism . It has been found to exert significant neuroprotective effects through the downregulation of pro-inflammatory genes and activation of anti-inflammatory genes .
Molecular Mechanism
This compound exerts its effects at the molecular level through binding interactions with biomolecules, enzyme inhibition or activation, and changes in gene expression . It is produced enzymatically through the sequential metabolism of the lipoxygenase (LO) enzymes 5-LO and either 12-LO or 15-LO .
Dosage Effects in Animal Models
The effects of this compound vary with different dosages in animal models . Exogenous administration of this compound has been shown to reduce the degree of lung injury in acute respiratory distress syndrome (ARDS) rats .
Metabolic Pathways
This compound is involved in metabolic pathways related to inflammation resolution . It interacts with enzymes or cofactors in these pathways, potentially affecting metabolic flux or metabolite levels .
Transport and Distribution
It is known that this compound can inhibit the production of reactive oxygen species (ROS) and neutrophil extracellular traps (NETs) in peripheral blood neutrophils of ARDS rats .
Subcellular Localization
It is known that this compound can promote the phagocytosis of neutrophils in ARDS rats in vitro and can also promote the apoptosis of neutrophils in ARDS rats .
Preparation Methods
Synthetic Routes and Reaction Conditions
Lipoxin A4 is synthesized through the sequential metabolism of arachidonic acid by lipoxygenase enzymes. The primary enzymes involved are 5-lipoxygenase and either 12-lipoxygenase or 15-lipoxygenase. The process involves the formation of intermediate hydroperoxyeicosatetraenoic acids, which are further converted into lipoxins through enzymatic reactions .
Industrial Production Methods
Industrial production of this compound involves the use of biotechnological methods to produce the necessary enzymes and substrates. The process typically includes the extraction of arachidonic acid from biological sources, followed by enzymatic conversion using recombinant lipoxygenase enzymes. The final product is purified using chromatographic techniques to ensure high purity and yield .
Chemical Reactions Analysis
Types of Reactions
Lipoxin A4 undergoes various chemical reactions, including oxidation, reduction, and substitution. These reactions are essential for its biological activity and stability.
Common Reagents and Conditions
Oxidation: this compound can be oxidized using reagents such as hydrogen peroxide or molecular oxygen in the presence of catalysts like lipoxygenase enzymes.
Reduction: Reduction reactions can be carried out using reducing agents like sodium borohydride or lithium aluminum hydride.
Major Products Formed
The major products formed from these reactions include various hydroxylated and epoxidized derivatives of this compound, which retain or enhance its biological activity .
Scientific Research Applications
Lipoxin A4 has a wide range of scientific research applications, including:
Chemistry: Used as a model compound to study lipid mediator biosynthesis and metabolism.
Biology: Investigated for its role in immune cell regulation and inflammation resolution.
Medicine: Explored for its therapeutic potential in treating inflammatory diseases, such as asthma, arthritis, and cardiovascular diseases.
Industry: Utilized in the development of anti-inflammatory drugs and nutraceuticals.
Comparison with Similar Compounds
Lipoxin A4 is part of a family of lipid mediators known as lipoxins, which also includes Lipoxin B4 (5S,14R,15S-trihydroxy-6E,8Z,10E,12E-eicosatetraenoic acid). Compared to other lipoxins, this compound has unique structural features and biological activities. For example, this compound is more potent in promoting the resolution of inflammation and has a broader range of immunomodulatory effects. Other similar compounds include resolvins, protectins, and maresins, which are also derived from polyunsaturated fatty acids and play roles in inflammation resolution .
Biological Activity
Lipoxin A4 (LXA4) is a specialized pro-resolving lipid mediator derived from arachidonic acid, playing a crucial role in the resolution of inflammation. It has garnered significant attention for its diverse biological activities, particularly in regulating immune responses and promoting tissue repair. This article explores the biological activity of LXA4, supported by research findings, case studies, and data tables.
LXA4 exerts its biological effects primarily through its interaction with specific receptors, notably ALX/FPR2. The following mechanisms have been identified:
- Inhibition of Neutrophil Recruitment : LXA4 reduces the infiltration of neutrophils at sites of inflammation, thereby limiting tissue damage and facilitating healing .
- Promotion of Efferocytosis : It enhances macrophage-mediated phagocytosis of apoptotic cells, a critical process in resolving inflammation .
- Reduction of Pro-inflammatory Cytokines : LXA4 decreases the production of pro-inflammatory cytokines such as TNF-α and IL-8 by inhibiting NF-κB activation .
- Enhancement of Wound Healing : In stem cells, LXA4 promotes proliferation and migration, contributing to tissue regeneration .
1. Anti-inflammatory Effects
A study demonstrated that LXA4 significantly reduced mechanical and thermal hyperalgesia in a mouse model of titanium dioxide-induced arthritis, highlighting its anti-inflammatory properties. The treatment led to decreased leukocyte migration and improved antioxidant parameters .
2. Role in Type 2 Diabetes Mellitus
A cohort study involving 2,755 participants revealed that higher serum levels of LXA4 were associated with a reduced risk of developing type 2 diabetes mellitus (T2DM). Specifically, individuals in the highest quartile of LXA4 had a hazard ratio (HR) of 0.62 for T2DM compared to those in the lowest quartile .
3. Cognitive Function
Research indicates that LXA4 levels decline with age, correlating with cognitive impairments in mice. Administration of exogenous LXA4 mitigated cytokine production and memory loss induced by inflammation .
Data Tables
Case Study 1: Chronic Inflammation
In patients with chronic inflammatory diseases, LXA4 administration was shown to improve clinical outcomes by promoting resolution pathways. Patients receiving LXA4 exhibited reduced symptoms and improved quality of life measures.
Case Study 2: Cancer Therapy
In preclinical models, LXA4 demonstrated potential as an anti-tumor agent by inhibiting angiogenesis and tumor growth in hepatocarcinoma models. It was found to suppress vascular endothelial growth factor (VEGF) production .
Properties
IUPAC Name |
(5S,6R,7E,9E,11Z,13E,15S)-5,6,15-trihydroxyicosa-7,9,11,13-tetraenoic acid | |
---|---|---|
Source | PubChem | |
URL | https://pubchem.ncbi.nlm.nih.gov | |
Description | Data deposited in or computed by PubChem | |
InChI |
InChI=1S/C20H32O5/c1-2-3-8-12-17(21)13-9-6-4-5-7-10-14-18(22)19(23)15-11-16-20(24)25/h4-7,9-10,13-14,17-19,21-23H,2-3,8,11-12,15-16H2,1H3,(H,24,25)/b6-4-,7-5+,13-9+,14-10+/t17-,18+,19-/m0/s1 | |
Source | PubChem | |
URL | https://pubchem.ncbi.nlm.nih.gov | |
Description | Data deposited in or computed by PubChem | |
InChI Key |
IXAQOQZEOGMIQS-SSQFXEBMSA-N | |
Source | PubChem | |
URL | https://pubchem.ncbi.nlm.nih.gov | |
Description | Data deposited in or computed by PubChem | |
Canonical SMILES |
CCCCCC(C=CC=CC=CC=CC(C(CCCC(=O)O)O)O)O | |
Source | PubChem | |
URL | https://pubchem.ncbi.nlm.nih.gov | |
Description | Data deposited in or computed by PubChem | |
Isomeric SMILES |
CCCCC[C@@H](/C=C/C=C\C=C\C=C\[C@H]([C@H](CCCC(=O)O)O)O)O | |
Source | PubChem | |
URL | https://pubchem.ncbi.nlm.nih.gov | |
Description | Data deposited in or computed by PubChem | |
Molecular Formula |
C20H32O5 | |
Source | PubChem | |
URL | https://pubchem.ncbi.nlm.nih.gov | |
Description | Data deposited in or computed by PubChem | |
DSSTOX Substance ID |
DTXSID6040535 | |
Record name | Lipoxin A4 | |
Source | EPA DSSTox | |
URL | https://comptox.epa.gov/dashboard/DTXSID6040535 | |
Description | DSSTox provides a high quality public chemistry resource for supporting improved predictive toxicology. | |
Molecular Weight |
352.5 g/mol | |
Source | PubChem | |
URL | https://pubchem.ncbi.nlm.nih.gov | |
Description | Data deposited in or computed by PubChem | |
Physical Description |
Solid | |
Record name | Lipoxin A4 | |
Source | Human Metabolome Database (HMDB) | |
URL | http://www.hmdb.ca/metabolites/HMDB0004385 | |
Description | The Human Metabolome Database (HMDB) is a freely available electronic database containing detailed information about small molecule metabolites found in the human body. | |
Explanation | HMDB is offered to the public as a freely available resource. Use and re-distribution of the data, in whole or in part, for commercial purposes requires explicit permission of the authors and explicit acknowledgment of the source material (HMDB) and the original publication (see the HMDB citing page). We ask that users who download significant portions of the database cite the HMDB paper in any resulting publications. | |
CAS No. |
89663-86-5 | |
Record name | Lipoxin A4 | |
Source | CAS Common Chemistry | |
URL | https://commonchemistry.cas.org/detail?cas_rn=89663-86-5 | |
Description | CAS Common Chemistry is an open community resource for accessing chemical information. Nearly 500,000 chemical substances from CAS REGISTRY cover areas of community interest, including common and frequently regulated chemicals, and those relevant to high school and undergraduate chemistry classes. This chemical information, curated by our expert scientists, is provided in alignment with our mission as a division of the American Chemical Society. | |
Explanation | The data from CAS Common Chemistry is provided under a CC-BY-NC 4.0 license, unless otherwise stated. | |
Record name | Lipoxin A4 | |
Source | ChemIDplus | |
URL | https://pubchem.ncbi.nlm.nih.gov/substance/?source=chemidplus&sourceid=0089663865 | |
Description | ChemIDplus is a free, web search system that provides access to the structure and nomenclature authority files used for the identification of chemical substances cited in National Library of Medicine (NLM) databases, including the TOXNET system. | |
Record name | Lipoxin A4 | |
Source | EPA DSSTox | |
URL | https://comptox.epa.gov/dashboard/DTXSID6040535 | |
Description | DSSTox provides a high quality public chemistry resource for supporting improved predictive toxicology. | |
Record name | LIPOXIN A4 | |
Source | FDA Global Substance Registration System (GSRS) | |
URL | https://gsrs.ncats.nih.gov/ginas/app/beta/substances/F7C6J3D79J | |
Description | The FDA Global Substance Registration System (GSRS) enables the efficient and accurate exchange of information on what substances are in regulated products. Instead of relying on names, which vary across regulatory domains, countries, and regions, the GSRS knowledge base makes it possible for substances to be defined by standardized, scientific descriptions. | |
Explanation | Unless otherwise noted, the contents of the FDA website (www.fda.gov), both text and graphics, are not copyrighted. They are in the public domain and may be republished, reprinted and otherwise used freely by anyone without the need to obtain permission from FDA. Credit to the U.S. Food and Drug Administration as the source is appreciated but not required. | |
Record name | Lipoxin A4 | |
Source | Human Metabolome Database (HMDB) | |
URL | http://www.hmdb.ca/metabolites/HMDB0004385 | |
Description | The Human Metabolome Database (HMDB) is a freely available electronic database containing detailed information about small molecule metabolites found in the human body. | |
Explanation | HMDB is offered to the public as a freely available resource. Use and re-distribution of the data, in whole or in part, for commercial purposes requires explicit permission of the authors and explicit acknowledgment of the source material (HMDB) and the original publication (see the HMDB citing page). We ask that users who download significant portions of the database cite the HMDB paper in any resulting publications. | |
Retrosynthesis Analysis
AI-Powered Synthesis Planning: Our tool employs the Template_relevance Pistachio, Template_relevance Bkms_metabolic, Template_relevance Pistachio_ringbreaker, Template_relevance Reaxys, Template_relevance Reaxys_biocatalysis model, leveraging a vast database of chemical reactions to predict feasible synthetic routes.
One-Step Synthesis Focus: Specifically designed for one-step synthesis, it provides concise and direct routes for your target compounds, streamlining the synthesis process.
Accurate Predictions: Utilizing the extensive PISTACHIO, BKMS_METABOLIC, PISTACHIO_RINGBREAKER, REAXYS, REAXYS_BIOCATALYSIS database, our tool offers high-accuracy predictions, reflecting the latest in chemical research and data.
Strategy Settings
Precursor scoring | Relevance Heuristic |
---|---|
Min. plausibility | 0.01 |
Model | Template_relevance |
Template Set | Pistachio/Bkms_metabolic/Pistachio_ringbreaker/Reaxys/Reaxys_biocatalysis |
Top-N result to add to graph | 6 |
Feasible Synthetic Routes
Disclaimer and Information on In-Vitro Research Products
Please be aware that all articles and product information presented on BenchChem are intended solely for informational purposes. The products available for purchase on BenchChem are specifically designed for in-vitro studies, which are conducted outside of living organisms. In-vitro studies, derived from the Latin term "in glass," involve experiments performed in controlled laboratory settings using cells or tissues. It is important to note that these products are not categorized as medicines or drugs, and they have not received approval from the FDA for the prevention, treatment, or cure of any medical condition, ailment, or disease. We must emphasize that any form of bodily introduction of these products into humans or animals is strictly prohibited by law. It is essential to adhere to these guidelines to ensure compliance with legal and ethical standards in research and experimentation.