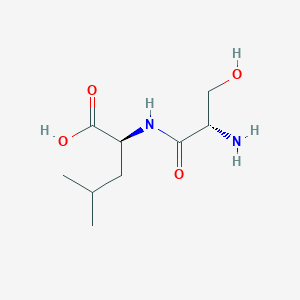
Ser-Leu
Overview
Description
Mechanism of Action
Target of Action
Seryl-Leucine, also known as Ser-Leu, is a dipeptide composed of the amino acids serine and leucine It’s worth noting that both serine and leucine are essential components of proteins and play crucial roles in various biological processes .
Mode of Action
The mode of action of this compound is complex and multifaceted, largely due to the diverse roles of its constituent amino acids, serine and leucine. For instance, in certain fungi like Candida albicans, a novel serine tRNA has been identified that can recognize the leucine CUG codon as serine . This unusual serine tRNA can be aminoacylated either with serine or leucine, leading to the incorporation of either of these amino acids at the CUG-encoded positions in proteins .
Biochemical Pathways
The biochemical pathways affected by this compound are primarily those involving protein synthesis and metabolism. The presence of serine and leucine in this dipeptide suggests it may influence pathways related to the metabolism of these amino acids. For instance, leucine is known to stimulate protein synthesis by activating the mTORC1 signaling pathway .
Result of Action
The molecular and cellular effects of this compound’s action are likely to be diverse, given the wide range of functions associated with serine and leucine. For example, leucine has been shown to promote muscle growth and metabolic health in animals and humans . On the other hand, serine plays a crucial role in the biosynthesis of purines and pyrimidines, essential components of DNA and RNA.
Action Environment
The action, efficacy, and stability of this compound can be influenced by various environmental factors. For instance, the ambiguous decoding of the leucine CUG codon as serine in certain fungi can lead to significant phenotypic alterations . Moreover, the effectiveness of leucine in promoting muscle protein synthesis and lean body mass accretion can be influenced by factors such as diet and exercise .
Biochemical Analysis
Biochemical Properties
Seryl-Leucine interacts with a variety of enzymes, proteins, and other biomolecules. For instance, it is recognized by aminoacyl-tRNA synthetases (aaRSs), which are crucial for protein synthesis . The interaction between Seryl-Leucine and these enzymes is essential for the selective quantification of amino acids, which is vital for clinical diagnostics .
Cellular Effects
Seryl-Leucine influences various cellular processes. For example, it has been identified as a metabolite in human urine, and its levels have been associated with bacterial load and inflammation in patients being treated for tuberculosis (TB) . This suggests that Seryl-Leucine may have a significant impact on cell signaling pathways, gene expression, and cellular metabolism.
Molecular Mechanism
At the molecular level, Seryl-Leucine exerts its effects through binding interactions with biomolecules and changes in gene expression. For instance, it interacts with aaRSs, leading to the activation of these enzymes and facilitating the process of protein synthesis .
Temporal Effects in Laboratory Settings
In laboratory settings, the effects of Seryl-Leucine can change over time. For example, in the context of TB treatment, the levels of Seryl-Leucine in urine decrease in association with a reduction of bacterial load and inflammation . This indicates the potential stability and long-term effects of Seryl-Leucine on cellular function.
Metabolic Pathways
Seryl-Leucine is involved in various metabolic pathways. It interacts with aaRSs, which play a crucial role in the metabolism of amino acids . Detailed information about the specific metabolic pathways that Seryl-Leucine is involved in is currently limited.
Preparation Methods
Synthetic Routes and Reaction Conditions
The synthesis of Serine-Leucine can be achieved through standard peptide synthesis techniques. One common method is solid-phase peptide synthesis (SPPS), which involves the stepwise addition of amino acids to a growing peptide chain anchored to a solid resin. The process typically includes the following steps:
Attachment of the first amino acid (serine) to the resin: The carboxyl group of serine is activated and coupled to the resin.
Deprotection: The protecting group on the amino group of serine is removed.
Coupling of the second amino acid (leucine): The carboxyl group of leucine is activated and coupled to the free amino group of serine.
Cleavage and purification: The dipeptide is cleaved from the resin and purified using techniques such as high-performance liquid chromatography (HPLC).
Industrial Production Methods
Industrial production of Serine-Leucine may involve large-scale SPPS or solution-phase peptide synthesis. In solution-phase synthesis, the amino acids are coupled in solution rather than on a solid support. This method can be more suitable for large-scale production but may require additional purification steps to remove by-products and unreacted starting materials.
Chemical Reactions Analysis
Types of Reactions
Serine-Leucine can undergo various chemical reactions, including:
Oxidation: The hydroxyl group of serine can be oxidized to form a ketone or aldehyde.
Reduction: The carbonyl group of the peptide bond can be reduced to form an amine.
Substitution: The hydroxyl group of serine can participate in nucleophilic substitution reactions.
Common Reagents and Conditions
Oxidation: Reagents such as hydrogen peroxide or potassium permanganate can be used under acidic or basic conditions.
Reduction: Reducing agents like sodium borohydride or lithium aluminum hydride can be employed.
Substitution: Nucleophiles such as halides or thiols can react with the hydroxyl group of serine.
Major Products
Oxidation: Oxidized derivatives of serine, such as serine aldehyde or serine ketone.
Reduction: Reduced forms of the peptide bond, resulting in secondary amines.
Substitution: Substituted derivatives of serine, depending on the nucleophile used.
Scientific Research Applications
Serine-Leucine has various applications in scientific research:
Chemistry: Used as a model compound to study peptide bond formation and cleavage.
Biology: Investigated for its role in protein-protein interactions and enzyme activity regulation.
Medicine: Explored for its potential therapeutic effects, such as modulating metabolic pathways or acting as a bioactive peptide.
Industry: Utilized in the development of peptide-based materials and as a building block for more complex peptides and proteins.
Comparison with Similar Compounds
Similar Compounds
Serine-Alanine: Another dipeptide with different biochemical properties due to the presence of alanine, a non-polar amino acid.
Leucine-Glycine: A dipeptide with glycine, the simplest amino acid, which can result in different structural and functional characteristics.
Uniqueness of Serine-Leucine
Serine-Leucine is unique due to the combination of a polar amino acid (serine) and a non-polar amino acid (leucine). This combination can result in distinct interactions with proteins and enzymes, making it a valuable compound for studying protein structure and function.
Properties
IUPAC Name |
(2S)-2-[[(2S)-2-amino-3-hydroxypropanoyl]amino]-4-methylpentanoic acid | |
---|---|---|
Details | Computed by LexiChem 2.6.6 (PubChem release 2019.06.18) | |
Source | PubChem | |
URL | https://pubchem.ncbi.nlm.nih.gov | |
Description | Data deposited in or computed by PubChem | |
InChI |
InChI=1S/C9H18N2O4/c1-5(2)3-7(9(14)15)11-8(13)6(10)4-12/h5-7,12H,3-4,10H2,1-2H3,(H,11,13)(H,14,15)/t6-,7-/m0/s1 | |
Details | Computed by InChI 1.0.5 (PubChem release 2019.06.18) | |
Source | PubChem | |
URL | https://pubchem.ncbi.nlm.nih.gov | |
Description | Data deposited in or computed by PubChem | |
InChI Key |
NFDYGNFETJVMSE-BQBZGAKWSA-N | |
Details | Computed by InChI 1.0.5 (PubChem release 2019.06.18) | |
Source | PubChem | |
URL | https://pubchem.ncbi.nlm.nih.gov | |
Description | Data deposited in or computed by PubChem | |
Canonical SMILES |
CC(C)CC(C(=O)O)NC(=O)C(CO)N | |
Details | Computed by OEChem 2.1.5 (PubChem release 2019.06.18) | |
Source | PubChem | |
URL | https://pubchem.ncbi.nlm.nih.gov | |
Description | Data deposited in or computed by PubChem | |
Isomeric SMILES |
CC(C)C[C@@H](C(=O)O)NC(=O)[C@H](CO)N | |
Details | Computed by OEChem 2.1.5 (PubChem release 2019.06.18) | |
Source | PubChem | |
URL | https://pubchem.ncbi.nlm.nih.gov | |
Description | Data deposited in or computed by PubChem | |
Molecular Formula |
C9H18N2O4 | |
Details | Computed by PubChem 2.1 (PubChem release 2019.06.18) | |
Source | PubChem | |
URL | https://pubchem.ncbi.nlm.nih.gov | |
Description | Data deposited in or computed by PubChem | |
Molecular Weight |
218.25 g/mol | |
Details | Computed by PubChem 2.1 (PubChem release 2021.05.07) | |
Source | PubChem | |
URL | https://pubchem.ncbi.nlm.nih.gov | |
Description | Data deposited in or computed by PubChem | |
Physical Description |
Solid | |
Record name | Serylleucine | |
Source | Human Metabolome Database (HMDB) | |
URL | http://www.hmdb.ca/metabolites/HMDB0029043 | |
Description | The Human Metabolome Database (HMDB) is a freely available electronic database containing detailed information about small molecule metabolites found in the human body. | |
Explanation | HMDB is offered to the public as a freely available resource. Use and re-distribution of the data, in whole or in part, for commercial purposes requires explicit permission of the authors and explicit acknowledgment of the source material (HMDB) and the original publication (see the HMDB citing page). We ask that users who download significant portions of the database cite the HMDB paper in any resulting publications. | |
CAS No. |
6665-16-3 | |
Record name | Serylleucine | |
Source | CAS Common Chemistry | |
URL | https://commonchemistry.cas.org/detail?cas_rn=6665-16-3 | |
Description | CAS Common Chemistry is an open community resource for accessing chemical information. Nearly 500,000 chemical substances from CAS REGISTRY cover areas of community interest, including common and frequently regulated chemicals, and those relevant to high school and undergraduate chemistry classes. This chemical information, curated by our expert scientists, is provided in alignment with our mission as a division of the American Chemical Society. | |
Explanation | The data from CAS Common Chemistry is provided under a CC-BY-NC 4.0 license, unless otherwise stated. | |
Record name | Serylleucine | |
Source | Human Metabolome Database (HMDB) | |
URL | http://www.hmdb.ca/metabolites/HMDB0029043 | |
Description | The Human Metabolome Database (HMDB) is a freely available electronic database containing detailed information about small molecule metabolites found in the human body. | |
Explanation | HMDB is offered to the public as a freely available resource. Use and re-distribution of the data, in whole or in part, for commercial purposes requires explicit permission of the authors and explicit acknowledgment of the source material (HMDB) and the original publication (see the HMDB citing page). We ask that users who download significant portions of the database cite the HMDB paper in any resulting publications. | |
Retrosynthesis Analysis
AI-Powered Synthesis Planning: Our tool employs the Template_relevance Pistachio, Template_relevance Bkms_metabolic, Template_relevance Pistachio_ringbreaker, Template_relevance Reaxys, Template_relevance Reaxys_biocatalysis model, leveraging a vast database of chemical reactions to predict feasible synthetic routes.
One-Step Synthesis Focus: Specifically designed for one-step synthesis, it provides concise and direct routes for your target compounds, streamlining the synthesis process.
Accurate Predictions: Utilizing the extensive PISTACHIO, BKMS_METABOLIC, PISTACHIO_RINGBREAKER, REAXYS, REAXYS_BIOCATALYSIS database, our tool offers high-accuracy predictions, reflecting the latest in chemical research and data.
Strategy Settings
Precursor scoring | Relevance Heuristic |
---|---|
Min. plausibility | 0.01 |
Model | Template_relevance |
Template Set | Pistachio/Bkms_metabolic/Pistachio_ringbreaker/Reaxys/Reaxys_biocatalysis |
Top-N result to add to graph | 6 |
Feasible Synthetic Routes
Disclaimer and Information on In-Vitro Research Products
Please be aware that all articles and product information presented on BenchChem are intended solely for informational purposes. The products available for purchase on BenchChem are specifically designed for in-vitro studies, which are conducted outside of living organisms. In-vitro studies, derived from the Latin term "in glass," involve experiments performed in controlled laboratory settings using cells or tissues. It is important to note that these products are not categorized as medicines or drugs, and they have not received approval from the FDA for the prevention, treatment, or cure of any medical condition, ailment, or disease. We must emphasize that any form of bodily introduction of these products into humans or animals is strictly prohibited by law. It is essential to adhere to these guidelines to ensure compliance with legal and ethical standards in research and experimentation.