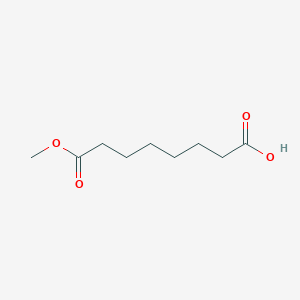
Suberic acid monomethyl ester
Overview
Description
It is a colorless solid with a melting point of 17-19°C and a boiling point of 185-186°C at 18 mmHg . This compound is a derivative of suberic acid, where one of the carboxyl groups is esterified with methanol.
Mechanism of Action
Target of Action
Suberic acid monomethyl ester, also known as monomethyl suberate, primarily targets the respiratory system
Mode of Action
It’s known that it has an effect onliver lactate metabolism
Biochemical Pathways
Given its impact on liver lactate metabolism, it can be inferred that it may influence pathways related tolactate metabolism
Result of Action
Given its known effect on liver lactate metabolism, it can be inferred that it may have a significant impact onliver function .
Preparation Methods
Synthetic Routes and Reaction Conditions: Suberic acid monomethyl ester is commonly synthesized through the esterification of suberic acid with methanol. The reaction typically occurs under acidic conditions using an acid catalyst such as sulfuric acid or a methylating agent like methylsulfuric acid . The general reaction is as follows:
HOOC-(CH2)6-COOH+CH3OH→HOOC-(CH2)6-COOCH3+H2O
Industrial Production Methods: In industrial settings, the esterification process is scaled up using continuous reactors to ensure efficient production. The reaction conditions are optimized to achieve high yields and purity. The product is then purified through distillation or recrystallization to remove any unreacted starting materials and by-products .
Chemical Reactions Analysis
Types of Reactions: Suberic acid monomethyl ester undergoes various chemical reactions, including:
Hydrolysis: The ester can be hydrolyzed back to suberic acid and methanol in the presence of water and an acid or base catalyst.
Reduction: It can be reduced to the corresponding alcohol using reducing agents like lithium aluminum hydride.
Substitution: The ester group can be substituted with other functional groups through nucleophilic substitution reactions.
Common Reagents and Conditions:
Hydrolysis: Acidic or basic conditions with water.
Reduction: Lithium aluminum hydride in anhydrous ether.
Substitution: Nucleophiles such as amines or alcohols under appropriate conditions.
Major Products:
Hydrolysis: Suberic acid and methanol.
Reduction: Suberic alcohol.
Substitution: Various substituted esters depending on the nucleophile used.
Scientific Research Applications
Suberic acid monomethyl ester has several applications in scientific research:
Comparison with Similar Compounds
Suberic acid monomethyl ester can be compared with other similar compounds such as:
Dimethyl suberate: Both are esters of suberic acid, but dimethyl suberate has two ester groups, making it more hydrophobic and less reactive in hydrolysis reactions.
Mono-methyl adipate: Similar in structure but derived from adipic acid, it has a shorter carbon chain, affecting its physical properties and reactivity.
Mono-methyl azelate: Another similar ester but derived from azelaic acid, it has a longer carbon chain, influencing its solubility and applications.
This compound stands out due to its balanced properties, making it versatile for various applications in research and industry.
Biological Activity
Suberic acid monomethyl ester, also known as monomethyl suberate, is an ester derived from suberic acid. It has gained attention for its potential biological activities, particularly in antifungal and antibacterial applications. This article reviews the current understanding of its biological activity, focusing on its antifungal properties, antibacterial effects, and molecular interactions.
Antifungal Activity
Research has shown that this compound exhibits moderate antifungal activity against various fungi. A study conducted by Antypenko et al. (2018) evaluated its effectiveness through growth inhibition assays involving 11 different fungal species and three strains of Phytophthora relevant to agriculture.
Key Findings:
- Concentration Range : The compound demonstrated antifungal effects at concentrations between 100–300 µg/mL.
- Comparison with Standards : When compared to hymexazol, a standard antifungal agent, this compound displayed similar but slightly lower efficacy.
- Molecular Docking Studies : In silico molecular docking suggested that monomethyl suberate interacts with several fungal enzymes, indicating potential mechanisms of action. The highest affinity was observed with N-myristoyltransferase (NMT), suggesting a possible target for its antifungal effects (affinity score of -6.0 kcal/mol) .
Fungal Target | Affinity Score (kcal/mol) |
---|---|
N-myristoyltransferase (NMT) | -6.0 |
Topoisomerase II | -5.5 |
Sterol 14α-demethylase (CYP51) | -5.3 |
UDP-N-acetyl-muramoyl-L-alanine: D-glutamate ligase (MurD) | -5.1 |
Secreted aspartic proteinase (SAP2) | -4.8 |
Antibacterial Activity
This compound has also been investigated for its antibacterial properties. A recent study highlighted its binding affinity to pathogenic proteins associated with Staphylococcus aureus and Escherichia coli, two major pathogens in bovine mastitis.
Key Findings:
- Binding Affinity : The compound showed significant binding affinities ranging from -5.0 to -5.9 kcal/mol against various bacterial proteins, including outer membrane protein A and Topoisomerase IV.
- Resistance Insights : The study indicated a concerning trend of increasing antimicrobial resistance in E. coli and S. aureus, emphasizing the need for novel antibacterial agents like this compound .
Bacterial Protein | Binding Affinity (kcal/mol) |
---|---|
Outer Membrane Protein A (E. coli) | -5.9 |
Topoisomerase IV (E. coli) | -5.0 |
Toxic Shock Syndrome Toxin-1 (S. aureus) | -5.1 |
Enterotoxin B (S. aureus) | -5.8 |
The mechanisms by which this compound exerts its biological activity are still under investigation. Molecular docking studies suggest that it may inhibit key enzymes involved in fungal cell wall synthesis and bacterial DNA replication.
Potential Targets:
- Fungal Enzymes : Inhibition of N-myristoyltransferase and other enzymes critical for fungal growth.
- Bacterial Proteins : Interaction with proteins involved in virulence and antibiotic resistance mechanisms.
Properties
IUPAC Name |
8-methoxy-8-oxooctanoic acid | |
---|---|---|
Source | PubChem | |
URL | https://pubchem.ncbi.nlm.nih.gov | |
Description | Data deposited in or computed by PubChem | |
InChI |
InChI=1S/C9H16O4/c1-13-9(12)7-5-3-2-4-6-8(10)11/h2-7H2,1H3,(H,10,11) | |
Source | PubChem | |
URL | https://pubchem.ncbi.nlm.nih.gov | |
Description | Data deposited in or computed by PubChem | |
InChI Key |
KOVPXZDUVJGGFU-UHFFFAOYSA-N | |
Source | PubChem | |
URL | https://pubchem.ncbi.nlm.nih.gov | |
Description | Data deposited in or computed by PubChem | |
Canonical SMILES |
COC(=O)CCCCCCC(=O)O | |
Source | PubChem | |
URL | https://pubchem.ncbi.nlm.nih.gov | |
Description | Data deposited in or computed by PubChem | |
Molecular Formula |
C9H16O4 | |
Source | PubChem | |
URL | https://pubchem.ncbi.nlm.nih.gov | |
Description | Data deposited in or computed by PubChem | |
DSSTOX Substance ID |
DTXSID90339174 | |
Record name | Suberic acid monomethyl ester | |
Source | EPA DSSTox | |
URL | https://comptox.epa.gov/dashboard/DTXSID90339174 | |
Description | DSSTox provides a high quality public chemistry resource for supporting improved predictive toxicology. | |
Molecular Weight |
188.22 g/mol | |
Source | PubChem | |
URL | https://pubchem.ncbi.nlm.nih.gov | |
Description | Data deposited in or computed by PubChem | |
CAS No. |
3946-32-5 | |
Record name | 1-Methyl octanedioate | |
Source | CAS Common Chemistry | |
URL | https://commonchemistry.cas.org/detail?cas_rn=3946-32-5 | |
Description | CAS Common Chemistry is an open community resource for accessing chemical information. Nearly 500,000 chemical substances from CAS REGISTRY cover areas of community interest, including common and frequently regulated chemicals, and those relevant to high school and undergraduate chemistry classes. This chemical information, curated by our expert scientists, is provided in alignment with our mission as a division of the American Chemical Society. | |
Explanation | The data from CAS Common Chemistry is provided under a CC-BY-NC 4.0 license, unless otherwise stated. | |
Record name | Suberic acid monomethyl ester | |
Source | EPA DSSTox | |
URL | https://comptox.epa.gov/dashboard/DTXSID90339174 | |
Description | DSSTox provides a high quality public chemistry resource for supporting improved predictive toxicology. | |
Retrosynthesis Analysis
AI-Powered Synthesis Planning: Our tool employs the Template_relevance Pistachio, Template_relevance Bkms_metabolic, Template_relevance Pistachio_ringbreaker, Template_relevance Reaxys, Template_relevance Reaxys_biocatalysis model, leveraging a vast database of chemical reactions to predict feasible synthetic routes.
One-Step Synthesis Focus: Specifically designed for one-step synthesis, it provides concise and direct routes for your target compounds, streamlining the synthesis process.
Accurate Predictions: Utilizing the extensive PISTACHIO, BKMS_METABOLIC, PISTACHIO_RINGBREAKER, REAXYS, REAXYS_BIOCATALYSIS database, our tool offers high-accuracy predictions, reflecting the latest in chemical research and data.
Strategy Settings
Precursor scoring | Relevance Heuristic |
---|---|
Min. plausibility | 0.01 |
Model | Template_relevance |
Template Set | Pistachio/Bkms_metabolic/Pistachio_ringbreaker/Reaxys/Reaxys_biocatalysis |
Top-N result to add to graph | 6 |
Feasible Synthetic Routes
Q1: What is the antifungal potential of Suberic acid monomethyl ester?
A1: Research suggests that this compound exhibits moderate antifungal activity against various fungi and oomycetes relevant to agriculture. Notably, it demonstrates significant inhibition against Alternaria alternata, Fusarium equiseti, Fusarium fujikuroi, and Phytophthora infestans GL-1 at concentrations ranging from 100-300 µg/mL. []
Q2: Which molecular target is most likely involved in the antifungal activity of this compound?
A2: While the precise mechanism of action remains under investigation, molecular docking studies indicate that this compound likely interacts with the N-myristoyltransferase enzyme in fungi. This enzyme plays a crucial role in fungal protein modification, and its inhibition could contribute to the observed antifungal effects. []
Q3: What is the role of this compound in synthesizing Hydroxamic acid-based Histone Deacetylase (HDAC) inhibitors?
A4: this compound serves as a key starting material in the multi-step synthesis of hydroxamic acid-based HDAC inhibitors like SAHA (Suberoylanilide hydroxamic acid). This compound acts as a building block, providing the suberic acid moiety present in the final HDAC inhibitor structure. []
Q4: Has this compound been identified in natural sources?
A5: Yes, this compound has been identified as one of the components of Acacia nilotica plant extracts. [] This finding suggests that this compound, in addition to its synthetic routes, might also be obtained from natural sources.
Q5: How does this compound interact with bacterial proteins?
A6: Molecular docking studies have shown that this compound exhibits binding affinity to specific proteins in both Escherichia coli and Staphylococcus aureus. In E. coli, it binds to outer membrane protein A and Topoisomerase IV. In S. aureus, it shows affinity for toxic shock syndrome toxin-1 and Enterotoxin B. These interactions suggest a potential antibacterial effect of the compound. []
Disclaimer and Information on In-Vitro Research Products
Please be aware that all articles and product information presented on BenchChem are intended solely for informational purposes. The products available for purchase on BenchChem are specifically designed for in-vitro studies, which are conducted outside of living organisms. In-vitro studies, derived from the Latin term "in glass," involve experiments performed in controlled laboratory settings using cells or tissues. It is important to note that these products are not categorized as medicines or drugs, and they have not received approval from the FDA for the prevention, treatment, or cure of any medical condition, ailment, or disease. We must emphasize that any form of bodily introduction of these products into humans or animals is strictly prohibited by law. It is essential to adhere to these guidelines to ensure compliance with legal and ethical standards in research and experimentation.