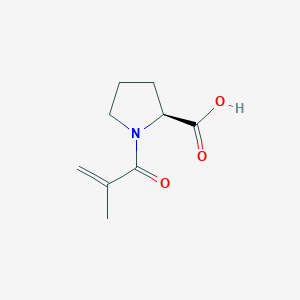
N-methacryloyl-L-proline
Overview
Description
1-(2-Methylacryloyl)-L-proline is an organic compound that belongs to the class of amino acid derivatives It is characterized by the presence of a proline moiety linked to a 2-methylacryloyl group
Mechanism of Action
Target of Action
N-methacryloyl-L-proline, also known as (2S)-1-(2-methylprop-2-enoyl)pyrrolidine-2-carboxylic acid or 1-(2-Methylacryloyl)-L-proline, is a biochemical used in proteomics research
Mode of Action
L-proline, a related compound, has been studied as a catalyst for the aldol condensation of formaldehyde and propionaldehyde to produce methacrolein . The catalytic activity of the reaction system was closely related to the competition between the main reaction synthesizing methacrolein and the side reaction producing 2-methyl-2-pentenal .
Result of Action
This compound has been used in the synthesis of peptidomimetic polymer brushes . These poly(this compound) grafted brush coatings are promising materials for numerous applications in nanomedicine, especially for the production of implants and systems of controlled interaction with proteins and cells .
Preparation Methods
Synthetic Routes and Reaction Conditions
The synthesis of 1-(2-Methylacryloyl)-L-proline typically involves the reaction of L-proline with 2-methylacryloyl chloride. The reaction is carried out in the presence of a base, such as triethylamine, to neutralize the hydrochloric acid formed during the reaction. The reaction is usually performed in an organic solvent like dichloromethane under an inert atmosphere to prevent unwanted side reactions .
Industrial Production Methods
Industrial production of 1-(2-Methylacryloyl)-L-proline can be achieved through continuous flow processes. These processes offer advantages such as improved reaction control, higher yields, and reduced formation of side products. The use of continuous flow reactors allows for the efficient and scalable production of this compound .
Chemical Reactions Analysis
Types of Reactions
1-(2-Methylacryloyl)-L-proline can undergo various chemical reactions, including:
Addition Reactions: The double bond in the 2-methylacryloyl group can participate in addition reactions with nucleophiles.
Substitution Reactions: The proline moiety can undergo substitution reactions, particularly at the amino group.
Common Reagents and Conditions
Addition Reactions: Common reagents include nucleophiles such as amines and thiols. The reactions are typically carried out under mild conditions.
Substitution Reactions: Reagents such as alkyl halides and acyl chlorides are used for substitution reactions at the amino group.
Polymerization: Radical initiators such as azobisisobutyronitrile (AIBN) are used to initiate polymerization reactions.
Major Products Formed
Addition Reactions: Products include adducts formed by the addition of nucleophiles to the double bond.
Substitution Reactions: Products include substituted proline derivatives.
Polymerization: Products include polymers with varying properties depending on the reaction conditions and monomers used.
Scientific Research Applications
1-(2-Methylacryloyl)-L-proline has several scientific research applications:
Chemistry: It is used as a building block for the synthesis of complex organic molecules and polymers.
Biology: It is studied for its potential role in biological systems, including its interactions with proteins and enzymes.
Medicine: It is investigated for its potential therapeutic applications, including drug delivery systems and as a component of biomaterials.
Industry: It is used in the production of advanced materials, including hydrogels and coatings.
Comparison with Similar Compounds
Similar Compounds
2-Methylacryloyl-L-alanine: Similar structure but with an alanine moiety instead of proline.
2-Methylacryloyl-L-valine: Similar structure but with a valine moiety instead of proline.
2-Methylacryloyl-L-leucine: Similar structure but with a leucine moiety instead of proline.
Uniqueness
1-(2-Methylacryloyl)-L-proline is unique due to the presence of the proline moiety, which imparts distinct structural and functional properties. The cyclic structure of proline can influence the reactivity and interactions of the compound, making it distinct from other similar compounds .
Biological Activity
N-methacryloyl-L-proline is a synthetic derivative of the naturally occurring amino acid L-proline. Its unique structure and properties have garnered attention for various biological applications, particularly in drug delivery systems, antimicrobial activity, and as a potential therapeutic agent in different diseases. This article explores the biological activity of this compound, supported by research findings, case studies, and data tables.
Chemical Structure and Properties
This compound is characterized by the presence of a methacryloyl group attached to the proline backbone. This modification enhances its reactivity and allows it to participate in various chemical reactions. The compound can form hydrogels that are biocompatible and suitable for drug delivery applications due to their ability to encapsulate therapeutic agents while minimizing systemic side effects.
1. Drug Delivery Systems
This compound has been utilized in the development of hydrogels that serve as drug delivery platforms. These hydrogels can be loaded with various drugs, including growth factors and antibiotics, allowing for localized and sustained release. In vitro studies have demonstrated that these hydrogels can maintain therapeutic drug levels over extended periods while exhibiting minimal cytotoxicity.
Table 1: Characteristics of this compound Hydrogels
Property | Value |
---|---|
Compressive Strength (MPa) | 195-210 |
Diametral Tensile Strength (MPa) | 19-26 |
Biaxial Flexural Strength (MPa) | 38-46 |
Drug Release Profile | Sustained over time |
2. Antimicrobial Properties
Research indicates that derivatives of L-proline, including this compound, possess antimicrobial properties. These compounds can inhibit the growth of various pathogens, which suggests their potential use as antimicrobial agents in medical applications .
3. Anti-inflammatory Effects
This compound has also been investigated for its anti-inflammatory properties. Studies suggest that proline derivatives can modulate inflammatory responses, making them candidates for therapeutic interventions in inflammatory diseases .
Case Studies
Case Study 1: Enhanced Mechanical Properties in Dental Applications
A study focused on incorporating this compound into glass ionomer cements (GICs) demonstrated significant improvements in mechanical properties compared to traditional GICs. The modified cements exhibited increased compressive strength (up to 170% higher), making them more durable for dental applications .
Case Study 2: Targeting Proline Uptake in Trypanosoma cruzi
In the context of Chagas disease, researchers explored the role of L-proline uptake by Trypanosoma cruzi. They developed inhibitors targeting proline transporters, which showed potential in disrupting the parasite's lifecycle and reducing its virulence. This approach highlights the significance of proline metabolism in pathogenic organisms and suggests that derivatives like this compound could be leveraged for therapeutic strategies against such infections .
While specific mechanisms of action for this compound remain under investigation, its structural similarity to natural amino acids allows it to participate in biochemical pathways relevant to cellular metabolism and signaling. The formation of amide bonds with other biomolecules may facilitate interactions that enhance its biological efficacy.
Properties
IUPAC Name |
(2S)-1-(2-methylprop-2-enoyl)pyrrolidine-2-carboxylic acid | |
---|---|---|
Source | PubChem | |
URL | https://pubchem.ncbi.nlm.nih.gov | |
Description | Data deposited in or computed by PubChem | |
InChI |
InChI=1S/C9H13NO3/c1-6(2)8(11)10-5-3-4-7(10)9(12)13/h7H,1,3-5H2,2H3,(H,12,13)/t7-/m0/s1 | |
Source | PubChem | |
URL | https://pubchem.ncbi.nlm.nih.gov | |
Description | Data deposited in or computed by PubChem | |
InChI Key |
SJAYUJDJZUWFDO-ZETCQYMHSA-N | |
Source | PubChem | |
URL | https://pubchem.ncbi.nlm.nih.gov | |
Description | Data deposited in or computed by PubChem | |
Canonical SMILES |
CC(=C)C(=O)N1CCCC1C(=O)O | |
Source | PubChem | |
URL | https://pubchem.ncbi.nlm.nih.gov | |
Description | Data deposited in or computed by PubChem | |
Isomeric SMILES |
CC(=C)C(=O)N1CCC[C@H]1C(=O)O | |
Source | PubChem | |
URL | https://pubchem.ncbi.nlm.nih.gov | |
Description | Data deposited in or computed by PubChem | |
Molecular Formula |
C9H13NO3 | |
Source | PubChem | |
URL | https://pubchem.ncbi.nlm.nih.gov | |
Description | Data deposited in or computed by PubChem | |
DSSTOX Substance ID |
DTXSID60527884 | |
Record name | 1-(2-Methylacryloyl)-L-proline | |
Source | EPA DSSTox | |
URL | https://comptox.epa.gov/dashboard/DTXSID60527884 | |
Description | DSSTox provides a high quality public chemistry resource for supporting improved predictive toxicology. | |
Molecular Weight |
183.20 g/mol | |
Source | PubChem | |
URL | https://pubchem.ncbi.nlm.nih.gov | |
Description | Data deposited in or computed by PubChem | |
CAS No. |
51161-88-7 | |
Record name | 1-(2-Methylacryloyl)-L-proline | |
Source | EPA DSSTox | |
URL | https://comptox.epa.gov/dashboard/DTXSID60527884 | |
Description | DSSTox provides a high quality public chemistry resource for supporting improved predictive toxicology. | |
Synthesis routes and methods
Procedure details
Q1: What are the potential applications of N-methacryloyl-L-proline in nanomedicine?
A: this compound exhibits promising potential in creating biocompatible polymeric nanolayers, particularly in the realm of nanomedicine. Research demonstrates that by grafting poly(this compound) brushes onto aminated glass surfaces, novel biocompatible coatings can be produced. [] These coatings hold significant promise for applications like implant development and the engineering of systems designed for controlled interactions with proteins and cells. []
Q2: How does the polymerization temperature affect the properties of poly(this compound)?
A: The polymerization temperature significantly influences the structural and optical properties of poly(this compound). Studies reveal that this compound exists in two isomeric forms: s-cis and s-trans. Higher polymerization temperatures favor the incorporation of the s-cis form into the polymer chain. [] Consequently, polymers synthesized at higher temperatures exhibit a higher s-cis content and display a lower absolute value of specific rotation, impacting their optical properties. []
Disclaimer and Information on In-Vitro Research Products
Please be aware that all articles and product information presented on BenchChem are intended solely for informational purposes. The products available for purchase on BenchChem are specifically designed for in-vitro studies, which are conducted outside of living organisms. In-vitro studies, derived from the Latin term "in glass," involve experiments performed in controlled laboratory settings using cells or tissues. It is important to note that these products are not categorized as medicines or drugs, and they have not received approval from the FDA for the prevention, treatment, or cure of any medical condition, ailment, or disease. We must emphasize that any form of bodily introduction of these products into humans or animals is strictly prohibited by law. It is essential to adhere to these guidelines to ensure compliance with legal and ethical standards in research and experimentation.