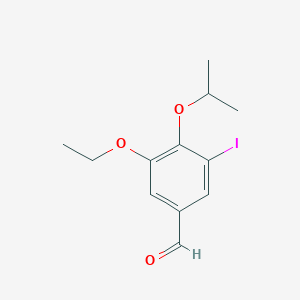
3-Ethoxy-5-iodo-4-isopropoxybenzaldehyde
Overview
Description
3-Ethoxy-5-iodo-4-isopropoxybenzaldehyde is a chemical compound with the molecular formula C12H15IO3 It is a benzaldehyde derivative, characterized by the presence of ethoxy, iodo, and isopropoxy groups attached to the benzene ring
Scientific Research Applications
3-Ethoxy-5-iodo-4-isopropoxybenzaldehyde has several applications in scientific research:
Chemistry: It serves as a building block for the synthesis of more complex organic molecules.
Biology: It is used in the study of biochemical pathways and enzyme interactions.
Industry: Utilized in the production of specialty chemicals and materials.
Preparation Methods
Synthetic Routes and Reaction Conditions
The synthesis of 3-Ethoxy-5-iodo-4-isopropoxybenzaldehyde typically involves multi-step organic reactions. One common method includes the iodination of 3-ethoxy-4-isopropoxybenzaldehyde using iodine and a suitable oxidizing agent. The reaction conditions often require a solvent such as acetic acid and a catalyst to facilitate the iodination process.
Industrial Production Methods
In an industrial setting, the production of this compound may involve large-scale batch reactions with optimized conditions to ensure high yield and purity. The process may include steps such as purification through recrystallization or chromatography to remove impurities and obtain the desired product.
Chemical Reactions Analysis
Types of Reactions
3-Ethoxy-5-iodo-4-isopropoxybenzaldehyde can undergo various chemical reactions, including:
Oxidation: The aldehyde group can be oxidized to form the corresponding carboxylic acid.
Reduction: The aldehyde group can be reduced to form the corresponding alcohol.
Substitution: The iodine atom can be substituted with other functional groups through nucleophilic substitution reactions.
Common Reagents and Conditions
Oxidation: Common oxidizing agents include potassium permanganate (KMnO4) and chromium trioxide (CrO3).
Reduction: Reducing agents such as sodium borohydride (NaBH4) or lithium aluminum hydride (LiAlH4) are typically used.
Substitution: Nucleophiles such as sodium azide (NaN3) or thiols (RSH) can be used for substitution reactions.
Major Products Formed
Oxidation: 3-Ethoxy-5-iodo-4-isopropoxybenzoic acid.
Reduction: 3-Ethoxy-5-iodo-4-isopropoxybenzyl alcohol.
Substitution: Various substituted derivatives depending on the nucleophile used.
Mechanism of Action
The mechanism of action of 3-Ethoxy-5-iodo-4-isopropoxybenzaldehyde involves its interaction with specific molecular targets, such as enzymes or receptors. The presence of the aldehyde group allows it to form covalent bonds with nucleophilic sites on proteins, potentially altering their function. The ethoxy, iodo, and isopropoxy groups contribute to the compound’s overall reactivity and specificity.
Comparison with Similar Compounds
Similar Compounds
- 3-Ethoxy-4-isopropoxybenzaldehyde
- 3-Iodo-4-isopropoxybenzaldehyde
- 3-Ethoxy-5-bromo-4-isopropoxybenzaldehyde
Uniqueness
3-Ethoxy-5-iodo-4-isopropoxybenzaldehyde is unique due to the combination of ethoxy, iodo, and isopropoxy groups, which confer distinct chemical properties and reactivity. This makes it a valuable compound for specific synthetic and research applications where these properties are desired.
Properties
IUPAC Name |
3-ethoxy-5-iodo-4-propan-2-yloxybenzaldehyde | |
---|---|---|
Details | Computed by LexiChem 2.6.6 (PubChem release 2019.06.18) | |
Source | PubChem | |
URL | https://pubchem.ncbi.nlm.nih.gov | |
Description | Data deposited in or computed by PubChem | |
InChI |
InChI=1S/C12H15IO3/c1-4-15-11-6-9(7-14)5-10(13)12(11)16-8(2)3/h5-8H,4H2,1-3H3 | |
Details | Computed by InChI 1.0.5 (PubChem release 2019.06.18) | |
Source | PubChem | |
URL | https://pubchem.ncbi.nlm.nih.gov | |
Description | Data deposited in or computed by PubChem | |
InChI Key |
YNSNCZFEJTXWIY-UHFFFAOYSA-N | |
Details | Computed by InChI 1.0.5 (PubChem release 2019.06.18) | |
Source | PubChem | |
URL | https://pubchem.ncbi.nlm.nih.gov | |
Description | Data deposited in or computed by PubChem | |
Canonical SMILES |
CCOC1=C(C(=CC(=C1)C=O)I)OC(C)C | |
Details | Computed by OEChem 2.1.5 (PubChem release 2019.06.18) | |
Source | PubChem | |
URL | https://pubchem.ncbi.nlm.nih.gov | |
Description | Data deposited in or computed by PubChem | |
Molecular Formula |
C12H15IO3 | |
Details | Computed by PubChem 2.1 (PubChem release 2019.06.18) | |
Source | PubChem | |
URL | https://pubchem.ncbi.nlm.nih.gov | |
Description | Data deposited in or computed by PubChem | |
Molecular Weight |
334.15 g/mol | |
Details | Computed by PubChem 2.1 (PubChem release 2021.05.07) | |
Source | PubChem | |
URL | https://pubchem.ncbi.nlm.nih.gov | |
Description | Data deposited in or computed by PubChem | |
Retrosynthesis Analysis
AI-Powered Synthesis Planning: Our tool employs the Template_relevance Pistachio, Template_relevance Bkms_metabolic, Template_relevance Pistachio_ringbreaker, Template_relevance Reaxys, Template_relevance Reaxys_biocatalysis model, leveraging a vast database of chemical reactions to predict feasible synthetic routes.
One-Step Synthesis Focus: Specifically designed for one-step synthesis, it provides concise and direct routes for your target compounds, streamlining the synthesis process.
Accurate Predictions: Utilizing the extensive PISTACHIO, BKMS_METABOLIC, PISTACHIO_RINGBREAKER, REAXYS, REAXYS_BIOCATALYSIS database, our tool offers high-accuracy predictions, reflecting the latest in chemical research and data.
Strategy Settings
Precursor scoring | Relevance Heuristic |
---|---|
Min. plausibility | 0.01 |
Model | Template_relevance |
Template Set | Pistachio/Bkms_metabolic/Pistachio_ringbreaker/Reaxys/Reaxys_biocatalysis |
Top-N result to add to graph | 6 |
Feasible Synthetic Routes
Disclaimer and Information on In-Vitro Research Products
Please be aware that all articles and product information presented on BenchChem are intended solely for informational purposes. The products available for purchase on BenchChem are specifically designed for in-vitro studies, which are conducted outside of living organisms. In-vitro studies, derived from the Latin term "in glass," involve experiments performed in controlled laboratory settings using cells or tissues. It is important to note that these products are not categorized as medicines or drugs, and they have not received approval from the FDA for the prevention, treatment, or cure of any medical condition, ailment, or disease. We must emphasize that any form of bodily introduction of these products into humans or animals is strictly prohibited by law. It is essential to adhere to these guidelines to ensure compliance with legal and ethical standards in research and experimentation.