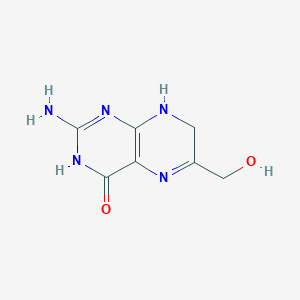
6-Hydroxymethyl-7,8-dihydropterin
Overview
Description
6-Hydroxymethyl-7,8-dihydropterin is a compound that plays a crucial role in the folate biosynthetic pathway. It serves as a precursor for several essential cofactors, including 5,6,7,8-tetrahydrofolate, methanopterin, and sarcinapterin. These cofactors are vital for various biological processes, such as nucleotide synthesis, amino acid metabolism, and DNA repair .
Synthesis Analysis
The biosynthesis of 6-Hydroxymethyl-7,8-dihydropterin involves several enzymatic steps. One of the key enzymes responsible for its production is 6-hydroxymethyl-dihydropterin diphosphate synthase . This enzyme catalyzes the conversion of GTP (guanosine triphosphate) into 6-Hydroxymethyl-7,8-dihydropterin diphosphate .
Molecular Structure Analysis
The molecular structure of 6-Hydroxymethyl-7,8-dihydropterin consists of a pterin ring system with a hydroxymethyl group attached at position 6. The chemical formula is C7H9N5O2 . The precise three-dimensional arrangement of atoms can be visualized through computational modeling techniques.
Chemical Reactions Analysis
The enzymatic reactions involving 6-Hydroxymethyl-7,8-dihydropterin are critical for the synthesis of folate derivatives. These reactions contribute to the production of essential cofactors required for cellular processes. Investigating the catalytic mechanisms and substrate interactions is essential for understanding its role in metabolism .
Scientific Research Applications
Chemical and Photochemical Reactivity
6-Hydroxymethyl-7,8-dihydropterin (H2Hmp) plays a crucial role as an intermediate in the biosynthesis of folate, which is essential in the metabolism of nucleotides and amino acids. A study by Thomas et al. (2013) explored its reactivity in aqueous solutions, revealing that H2Hmp undergoes thermal oxidation and can form a dimer upon UV-A excitation, indicating its potential for further chemical and photochemical research applications (Thomas et al., 2013).
Role in Folate Biosynthesis
Illarionova et al. (2002) described the biosynthesis of tetrahydrofolate, highlighting the formation of 6-hydroxymethyl-7,8-dihydropterin as a precursor in this pathway. This process is a potential target for antimicrobial and anti-parasite chemotherapy, underscoring its importance in medical research (Illarionova et al., 2002).
Inhibitor Design for Enzyme Targeting
The enzyme 6-Hydroxymethyl-7,8-dihydropterin pyrophosphokinase (HPPK), which catalyzes a step in the folate biosynthesis pathway, has been a focus for developing novel inhibitors. Dennis et al. (2016) demonstrated the synthesis of inhibitors that bind selectively to HPPK, presenting opportunities for antimicrobial agent development (Dennis et al., 2016).
Molecular Dynamics and Structural Analysis
Gao et al. (2016) conducted molecular dynamics simulations of HPPK with MgATP, revealing significant conformational changes in catalytic loops. This research offers insights into enzyme function and potential drug targeting (Gao et al., 2016).
Insights into Folate Synthesis in Plants
Mouillon et al. (2002) studied the coupling between reactions in tetrahydrofolate synthesis in plant mitochondria. Their work on 6-hydroxymethyl-7,8-dihydropterin pyrophosphokinase/7,8-dihydropteroate synthase (HPPK/DHPS) provides crucial insights into plant biochemistry and potential agricultural applications (Mouillon et al., 2002).
Development of Novel Antimicrobial Agents
Research by Yun et al. (2014) on identifying novel inhibitors of HPPK emphasizes the potential of 6-Hydroxymethyl-7,8-dihydropterin in developing new antimicrobial agents, especially in the context of increasing resistance to traditional drugs (Yun et al., 2014).
Mechanism of Action
6-Hydroxymethyl-7,8-dihydropterin participates in the biosynthesis of tetrahydrofolate (THF), a crucial cofactor involved in one-carbon transfer reactions. THF is essential for nucleotide synthesis, amino acid metabolism, and DNA synthesis. The enzyme HPPK (6-hydroxymethyl-7,8-dihydropterin pyrophosphokinase) plays a central role in converting 6-Hydroxymethyl-7,8-dihydropterin into its active form .
Future Directions
Research on 6-Hydroxymethyl-7,8-dihydropterin continues to explore its role in cellular metabolism, potential therapeutic applications, and drug development. Investigating its interactions with enzymes and inhibitors may lead to novel antimicrobial agents .
: Pemble CW IV, Mehta PK, Mehra S, Li Z, Nourse A, Lee RE, et al. (2010) Crystal Structure of the 6-Hydroxymethyl-7,8-Dihydropterin Pyrophosphokinase•Dihydropteroate Synthase Bifunctional Enzyme from Francisella tularensis. PLoS ONE 5 (11): e14165. Read more : MetaCyc Pathway: 6-hydroxymethyl-dihydropterin diphosphate biosynthesis I. Read more : Schircks Laboratories. Read more :
properties
IUPAC Name |
2-amino-6-(hydroxymethyl)-7,8-dihydro-3H-pteridin-4-one | |
---|---|---|
Details | Computed by LexiChem 2.6.6 (PubChem release 2019.06.18) | |
Source | PubChem | |
URL | https://pubchem.ncbi.nlm.nih.gov | |
Description | Data deposited in or computed by PubChem | |
InChI |
InChI=1S/C7H9N5O2/c8-7-11-5-4(6(14)12-7)10-3(2-13)1-9-5/h13H,1-2H2,(H4,8,9,11,12,14) | |
Details | Computed by InChI 1.0.5 (PubChem release 2019.06.18) | |
Source | PubChem | |
URL | https://pubchem.ncbi.nlm.nih.gov | |
Description | Data deposited in or computed by PubChem | |
InChI Key |
CQQNNQTXUGLUEV-UHFFFAOYSA-N | |
Details | Computed by InChI 1.0.5 (PubChem release 2019.06.18) | |
Source | PubChem | |
URL | https://pubchem.ncbi.nlm.nih.gov | |
Description | Data deposited in or computed by PubChem | |
Canonical SMILES |
C1C(=NC2=C(N1)N=C(NC2=O)N)CO | |
Details | Computed by OEChem 2.1.5 (PubChem release 2019.06.18) | |
Source | PubChem | |
URL | https://pubchem.ncbi.nlm.nih.gov | |
Description | Data deposited in or computed by PubChem | |
Molecular Formula |
C7H9N5O2 | |
Details | Computed by PubChem 2.1 (PubChem release 2019.06.18) | |
Source | PubChem | |
URL | https://pubchem.ncbi.nlm.nih.gov | |
Description | Data deposited in or computed by PubChem | |
DSSTOX Substance ID |
DTXSID00274264 | |
Record name | 6-HYDROXYMETHYL-7,8-DIHYDROPTERIN | |
Source | EPA DSSTox | |
URL | https://comptox.epa.gov/dashboard/DTXSID00274264 | |
Description | DSSTox provides a high quality public chemistry resource for supporting improved predictive toxicology. | |
Molecular Weight |
195.18 g/mol | |
Details | Computed by PubChem 2.1 (PubChem release 2021.05.07) | |
Source | PubChem | |
URL | https://pubchem.ncbi.nlm.nih.gov | |
Description | Data deposited in or computed by PubChem | |
Product Name |
6-Hydroxymethyl-7,8-dihydropterin | |
CAS RN |
3672-03-5 | |
Record name | 2-Amino-6-(hydroxymethyl)-7,8-dihydro-3H-pteridin-4-one | |
Source | ChemIDplus | |
URL | https://pubchem.ncbi.nlm.nih.gov/substance/?source=chemidplus&sourceid=0003672035 | |
Description | ChemIDplus is a free, web search system that provides access to the structure and nomenclature authority files used for the identification of chemical substances cited in National Library of Medicine (NLM) databases, including the TOXNET system. | |
Record name | 6-Hydroxymethyl-7,8-Dihydropterin | |
Source | DrugBank | |
URL | https://www.drugbank.ca/drugs/DB02119 | |
Description | The DrugBank database is a unique bioinformatics and cheminformatics resource that combines detailed drug (i.e. chemical, pharmacological and pharmaceutical) data with comprehensive drug target (i.e. sequence, structure, and pathway) information. | |
Explanation | Creative Common's Attribution-NonCommercial 4.0 International License (http://creativecommons.org/licenses/by-nc/4.0/legalcode) | |
Record name | 6-HYDROXYMETHYL-7,8-DIHYDROPTERIN | |
Source | EPA DSSTox | |
URL | https://comptox.epa.gov/dashboard/DTXSID00274264 | |
Description | DSSTox provides a high quality public chemistry resource for supporting improved predictive toxicology. | |
Record name | 2-AMINO-6-(HYDROXYMETHYL)-7,8-DIHYDRO-3H-PTERIDIN-4-ONE | |
Source | FDA Global Substance Registration System (GSRS) | |
URL | https://gsrs.ncats.nih.gov/ginas/app/beta/substances/B46L5LR2XM | |
Description | The FDA Global Substance Registration System (GSRS) enables the efficient and accurate exchange of information on what substances are in regulated products. Instead of relying on names, which vary across regulatory domains, countries, and regions, the GSRS knowledge base makes it possible for substances to be defined by standardized, scientific descriptions. | |
Explanation | Unless otherwise noted, the contents of the FDA website (www.fda.gov), both text and graphics, are not copyrighted. They are in the public domain and may be republished, reprinted and otherwise used freely by anyone without the need to obtain permission from FDA. Credit to the U.S. Food and Drug Administration as the source is appreciated but not required. | |
Retrosynthesis Analysis
AI-Powered Synthesis Planning: Our tool employs the Template_relevance Pistachio, Template_relevance Bkms_metabolic, Template_relevance Pistachio_ringbreaker, Template_relevance Reaxys, Template_relevance Reaxys_biocatalysis model, leveraging a vast database of chemical reactions to predict feasible synthetic routes.
One-Step Synthesis Focus: Specifically designed for one-step synthesis, it provides concise and direct routes for your target compounds, streamlining the synthesis process.
Accurate Predictions: Utilizing the extensive PISTACHIO, BKMS_METABOLIC, PISTACHIO_RINGBREAKER, REAXYS, REAXYS_BIOCATALYSIS database, our tool offers high-accuracy predictions, reflecting the latest in chemical research and data.
Strategy Settings
Precursor scoring | Relevance Heuristic |
---|---|
Min. plausibility | 0.01 |
Model | Template_relevance |
Template Set | Pistachio/Bkms_metabolic/Pistachio_ringbreaker/Reaxys/Reaxys_biocatalysis |
Top-N result to add to graph | 6 |
Feasible Synthetic Routes
Disclaimer and Information on In-Vitro Research Products
Please be aware that all articles and product information presented on BenchChem are intended solely for informational purposes. The products available for purchase on BenchChem are specifically designed for in-vitro studies, which are conducted outside of living organisms. In-vitro studies, derived from the Latin term "in glass," involve experiments performed in controlled laboratory settings using cells or tissues. It is important to note that these products are not categorized as medicines or drugs, and they have not received approval from the FDA for the prevention, treatment, or cure of any medical condition, ailment, or disease. We must emphasize that any form of bodily introduction of these products into humans or animals is strictly prohibited by law. It is essential to adhere to these guidelines to ensure compliance with legal and ethical standards in research and experimentation.