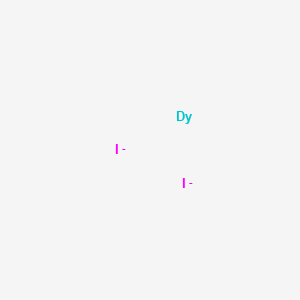
Dysprosium(II) iodide
Overview
Description
Dysprosium(II) iodide is a lanthanide salt . It is insoluble in water and is often used in the synthesis of fine chemicals . It is also used as a heat and light stabilizer for nylon fabrics .
Synthesis Analysis
Dysprosium(II) iodide can be produced by reducing dysprosium(III) iodide with metallic dysprosium under a vacuum at 800 to 900 °C . It can also be formed by the reaction of dysprosium and mercury(II) iodide . Another method of formation is the direct reaction of dysprosium and iodine .Molecular Structure Analysis
Dysprosium(II) iodide has the chemical formula DyI2 . Dysprosium (atomic symbol: Dy, atomic number: 66) is a Block F, Group 3, Period 6 element with an atomic radius of 162.5 . The number of electrons in each of dysprosium’s shells is [2, 8, 18, 28, 8, 2] and its electron configuration is [Xe]4f 10 6s 2 .Chemical Reactions Analysis
Dysprosium(II) iodide can react with silicon tetrachloride to produce trichlorosilyl radicals . These radicals can catalyze the cyclotrimerization of alkynes .Physical And Chemical Properties Analysis
Dysprosium(II) iodide is a dark purple-black solid . It is easily deliquescent and can only be stored in a dry inert gas or a vacuum . In the air, it will absorb moisture and become a hydrate, but they are unstable and will quickly convert into iodide oxides and release hydrogen gas . This process occurs faster in the presence of water . This compound has the same crystal structure as cadmium chloride . It can form complexes with tetrahydrofuran, butanol, and phenol .Scientific Research Applications
Synthesis and Stability
Dysprosium(II) iodide has been synthesized using a method involving heating with iodine, followed by dissolving in THF or DME and crystallization at low temperatures. This method has produced microcrystalline molecular iodides of dysprosium(II), which are thermally stable in their solid form, though less stable in solution (Bochkarev & Fagin, 1999).
Application in Spectrometry
A notable application of dysprosium in scientific research is in neutron activation and gamma-ray spectrometry. It has been used for the determination of scandium and dysprosium in various materials, such as rare earth-rich ores and yttrium oxide, demonstrating its utility in quantitative chemical analysis (Okada, 1961).
Influence in Arc Tubes
Research has shown that dysprosium-thallium and scandium-sodium iodide lamps, when operated horizontally, lead to silica transport within the arc tube. This process results in the etching of the internal surface and the precipitation of amorphous SiO2, impacting the lamp's performance (Yamane & Suenaga, 1983).
Luminescence in Molecular Compounds
Dysprosium(II) iodide has been used in the synthesis of unique molecular compounds with luminescent properties. These compounds exhibit metal-centered luminescence characteristic of the Dy(3+) ion, contributing to the field of photochemistry (Fagin et al., 2016).
Photoluminescence in Nanotechnology
Dysprosium-doped lead iodide nanostructures have been synthesized for potential optoelectronic applications. These nanostructures displayed varied photoluminescence emissions, highlighting their potential use in advanced technology applications (Chandekar et al., 2020).
Medical Research Applications
In the field of biology and medicine, dysprosium(III) complexes have been studied for their DNA binding properties and antimicrobial activity. This research has implications for the development of new therapeutic agents and diagnostic tools (Khorasani-Motlagh et al., 2013).
Implications in High-Intensity Discharge Lamps
Dysprosium iodide's use in high-intensity discharge lamps has been studied extensively. It is crucial for the lamps' efficiency and affects color separation due to axial segregation, which has implications in lighting technology (Flikweert et al., 2005).
Safety and Hazards
Dysprosium(II) iodide is classified as a skin irritant (Category 2), eye irritant (Category 2A), and may cause respiratory irritation (Specific target organ toxicity - single exposure, Category 3) . It is recommended to avoid breathing dust/fume/gas/mist/vapors/spray, and to avoid getting it in eyes, on skin, or on clothing . It should be used only outdoors or in a well-ventilated area, and protective gloves/eye protection/face protection should be worn .
properties
IUPAC Name |
dysprosium;diiodide | |
---|---|---|
Details | Computed by LexiChem 2.6.6 (PubChem release 2019.06.18) | |
Source | PubChem | |
URL | https://pubchem.ncbi.nlm.nih.gov | |
Description | Data deposited in or computed by PubChem | |
InChI |
InChI=1S/Dy.2HI/h;2*1H/p-2 | |
Details | Computed by InChI 1.0.5 (PubChem release 2019.06.18) | |
Source | PubChem | |
URL | https://pubchem.ncbi.nlm.nih.gov | |
Description | Data deposited in or computed by PubChem | |
InChI Key |
SSDFPWIJYIKUFK-UHFFFAOYSA-L | |
Details | Computed by InChI 1.0.5 (PubChem release 2019.06.18) | |
Source | PubChem | |
URL | https://pubchem.ncbi.nlm.nih.gov | |
Description | Data deposited in or computed by PubChem | |
Canonical SMILES |
[I-].[I-].[Dy] | |
Details | Computed by OEChem 2.1.5 (PubChem release 2019.06.18) | |
Source | PubChem | |
URL | https://pubchem.ncbi.nlm.nih.gov | |
Description | Data deposited in or computed by PubChem | |
Molecular Formula |
DyI2-2 | |
Details | Computed by PubChem 2.1 (PubChem release 2019.06.18) | |
Source | PubChem | |
URL | https://pubchem.ncbi.nlm.nih.gov | |
Description | Data deposited in or computed by PubChem | |
DSSTOX Substance ID |
DTXSID20849397 | |
Record name | dysprosium;diiodide | |
Source | EPA DSSTox | |
URL | https://comptox.epa.gov/dashboard/DTXSID20849397 | |
Description | DSSTox provides a high quality public chemistry resource for supporting improved predictive toxicology. | |
Molecular Weight |
416.309 g/mol | |
Details | Computed by PubChem 2.1 (PubChem release 2021.08.13) | |
Source | PubChem | |
URL | https://pubchem.ncbi.nlm.nih.gov | |
Description | Data deposited in or computed by PubChem | |
Product Name |
Dysprosium(II) iodide | |
CAS RN |
36377-94-3 | |
Record name | dysprosium;diiodide | |
Source | EPA DSSTox | |
URL | https://comptox.epa.gov/dashboard/DTXSID20849397 | |
Description | DSSTox provides a high quality public chemistry resource for supporting improved predictive toxicology. | |
Record name | Dysprosium(II) iodide | |
Source | European Chemicals Agency (ECHA) | |
URL | https://echa.europa.eu/information-on-chemicals | |
Description | The European Chemicals Agency (ECHA) is an agency of the European Union which is the driving force among regulatory authorities in implementing the EU's groundbreaking chemicals legislation for the benefit of human health and the environment as well as for innovation and competitiveness. | |
Explanation | Use of the information, documents and data from the ECHA website is subject to the terms and conditions of this Legal Notice, and subject to other binding limitations provided for under applicable law, the information, documents and data made available on the ECHA website may be reproduced, distributed and/or used, totally or in part, for non-commercial purposes provided that ECHA is acknowledged as the source: "Source: European Chemicals Agency, http://echa.europa.eu/". Such acknowledgement must be included in each copy of the material. ECHA permits and encourages organisations and individuals to create links to the ECHA website under the following cumulative conditions: Links can only be made to webpages that provide a link to the Legal Notice page. | |
Disclaimer and Information on In-Vitro Research Products
Please be aware that all articles and product information presented on BenchChem are intended solely for informational purposes. The products available for purchase on BenchChem are specifically designed for in-vitro studies, which are conducted outside of living organisms. In-vitro studies, derived from the Latin term "in glass," involve experiments performed in controlled laboratory settings using cells or tissues. It is important to note that these products are not categorized as medicines or drugs, and they have not received approval from the FDA for the prevention, treatment, or cure of any medical condition, ailment, or disease. We must emphasize that any form of bodily introduction of these products into humans or animals is strictly prohibited by law. It is essential to adhere to these guidelines to ensure compliance with legal and ethical standards in research and experimentation.