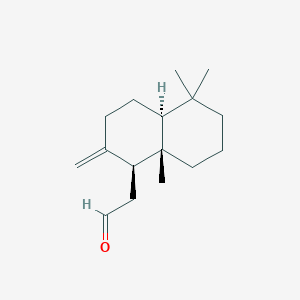
Bicyclohomofarnesal
Overview
Description
Preparation Methods
Bicyclohomofarnesal can be synthesized through a multi-step process starting from sclareol, a natural diterpene alcohol. The key steps in the synthesis include the ozonolytic cleavage of sclareol to form bis(8α,13-epoxy-14,15-bisnorlabd-12-en-12-yl)methane, followed by further ozonolysis and alkaline cleavage to yield this compound . The reaction conditions typically involve the use of methanol and anhydrous ammonium chloride, with the ozonolysis performed at low temperatures .
Chemical Reactions Analysis
Bicyclohomofarnesal undergoes various chemical reactions, including oxidation, reduction, and substitution reactions. Common reagents used in these reactions include oxidizing agents like potassium permanganate and reducing agents like sodium borohydride. The major products formed from these reactions depend on the specific conditions and reagents used. For example, oxidation of this compound can yield carboxylic acids, while reduction can produce alcohols .
Scientific Research Applications
Bicyclohomofarnesal has been extensively studied for its applications in various fields:
Chemistry: It is used as a synthetic intermediate in the production of other fragrance compounds.
Biology: Research has explored its potential as a bioactive compound with antimicrobial properties.
Medicine: Studies have investigated its potential therapeutic effects, including its use as an anti-inflammatory agent.
Mechanism of Action
The mechanism of action of bicyclohomofarnesal involves its interaction with olfactory receptors, which are responsible for detecting odors. The compound binds to these receptors, triggering a signal transduction pathway that results in the perception of its characteristic ambergris-like odor. Additionally, its antimicrobial and anti-inflammatory effects are believed to be mediated through interactions with specific molecular targets and pathways, although the exact mechanisms are still under investigation .
Comparison with Similar Compounds
Bicyclohomofarnesal is unique among similar compounds due to its distinct ambergris-like odor and its synthetic origin. Similar compounds include:
Ambroxide: Another synthetic fragrance compound with a similar odor profile.
Sclareolide: A natural compound derived from sclareol, used in the fragrance industry.
Iso E Super: A synthetic fragrance compound with a woody, amber-like scent.
This compound stands out due to its specific synthesis route and its widespread use in the fragrance industry.
Properties
IUPAC Name |
2-[(1S,4aS,8aS)-5,5,8a-trimethyl-2-methylidene-3,4,4a,6,7,8-hexahydro-1H-naphthalen-1-yl]acetaldehyde | |
---|---|---|
Details | Computed by LexiChem 2.6.6 (PubChem release 2019.06.18) | |
Source | PubChem | |
URL | https://pubchem.ncbi.nlm.nih.gov | |
Description | Data deposited in or computed by PubChem | |
InChI |
InChI=1S/C16H26O/c1-12-6-7-14-15(2,3)9-5-10-16(14,4)13(12)8-11-17/h11,13-14H,1,5-10H2,2-4H3/t13-,14-,16+/m0/s1 | |
Details | Computed by InChI 1.0.5 (PubChem release 2019.06.18) | |
Source | PubChem | |
URL | https://pubchem.ncbi.nlm.nih.gov | |
Description | Data deposited in or computed by PubChem | |
InChI Key |
BFWKKBSHTOEBHL-OFQRWUPVSA-N | |
Details | Computed by InChI 1.0.5 (PubChem release 2019.06.18) | |
Source | PubChem | |
URL | https://pubchem.ncbi.nlm.nih.gov | |
Description | Data deposited in or computed by PubChem | |
Canonical SMILES |
CC1(CCCC2(C1CCC(=C)C2CC=O)C)C | |
Details | Computed by OEChem 2.1.5 (PubChem release 2019.06.18) | |
Source | PubChem | |
URL | https://pubchem.ncbi.nlm.nih.gov | |
Description | Data deposited in or computed by PubChem | |
Isomeric SMILES |
C[C@]12CCCC([C@@H]1CCC(=C)[C@@H]2CC=O)(C)C | |
Details | Computed by OEChem 2.1.5 (PubChem release 2019.06.18) | |
Source | PubChem | |
URL | https://pubchem.ncbi.nlm.nih.gov | |
Description | Data deposited in or computed by PubChem | |
Molecular Formula |
C16H26O | |
Details | Computed by PubChem 2.1 (PubChem release 2019.06.18) | |
Source | PubChem | |
URL | https://pubchem.ncbi.nlm.nih.gov | |
Description | Data deposited in or computed by PubChem | |
Molecular Weight |
234.38 g/mol | |
Details | Computed by PubChem 2.1 (PubChem release 2021.05.07) | |
Source | PubChem | |
URL | https://pubchem.ncbi.nlm.nih.gov | |
Description | Data deposited in or computed by PubChem | |
Retrosynthesis Analysis
AI-Powered Synthesis Planning: Our tool employs the Template_relevance Pistachio, Template_relevance Bkms_metabolic, Template_relevance Pistachio_ringbreaker, Template_relevance Reaxys, Template_relevance Reaxys_biocatalysis model, leveraging a vast database of chemical reactions to predict feasible synthetic routes.
One-Step Synthesis Focus: Specifically designed for one-step synthesis, it provides concise and direct routes for your target compounds, streamlining the synthesis process.
Accurate Predictions: Utilizing the extensive PISTACHIO, BKMS_METABOLIC, PISTACHIO_RINGBREAKER, REAXYS, REAXYS_BIOCATALYSIS database, our tool offers high-accuracy predictions, reflecting the latest in chemical research and data.
Strategy Settings
Precursor scoring | Relevance Heuristic |
---|---|
Min. plausibility | 0.01 |
Model | Template_relevance |
Template Set | Pistachio/Bkms_metabolic/Pistachio_ringbreaker/Reaxys/Reaxys_biocatalysis |
Top-N result to add to graph | 6 |
Feasible Synthetic Routes
Disclaimer and Information on In-Vitro Research Products
Please be aware that all articles and product information presented on BenchChem are intended solely for informational purposes. The products available for purchase on BenchChem are specifically designed for in-vitro studies, which are conducted outside of living organisms. In-vitro studies, derived from the Latin term "in glass," involve experiments performed in controlled laboratory settings using cells or tissues. It is important to note that these products are not categorized as medicines or drugs, and they have not received approval from the FDA for the prevention, treatment, or cure of any medical condition, ailment, or disease. We must emphasize that any form of bodily introduction of these products into humans or animals is strictly prohibited by law. It is essential to adhere to these guidelines to ensure compliance with legal and ethical standards in research and experimentation.