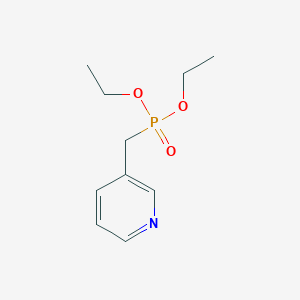
3-(Diethoxyphosphorylmethyl)pyridine
Overview
Description
3-(Diethoxyphosphorylmethyl)pyridine is an organic compound with the molecular formula C10H16NO3P. It is a derivative of pyridine, a six-membered aromatic heterocycle containing one nitrogen atom. The compound features a diethoxyphosphorylmethyl group attached to the third position of the pyridine ring. This functional group imparts unique chemical properties to the molecule, making it valuable in various scientific and industrial applications.
Preparation Methods
Synthetic Routes and Reaction Conditions
The synthesis of 3-(Diethoxyphosphorylmethyl)pyridine typically involves the reaction of pyridine with diethyl phosphite in the presence of a base. One common method is the Michaelis-Arbuzov reaction, where pyridine is treated with diethyl phosphite under basic conditions to yield the desired product. The reaction is usually carried out at elevated temperatures to ensure complete conversion.
Industrial Production Methods
In an industrial setting, the production of this compound can be scaled up by optimizing the reaction conditions, such as temperature, pressure, and the concentration of reactants. Continuous flow reactors may be employed to enhance the efficiency and yield of the process. Additionally, purification techniques like distillation and crystallization are used to obtain the compound in high purity.
Chemical Reactions Analysis
Types of Reactions
3-(Diethoxyphosphorylmethyl)pyridine undergoes various chemical reactions, including:
Oxidation: The compound can be oxidized to form corresponding phosphonic acids or phosphonates.
Reduction: Reduction reactions can convert the phosphoryl group to a phosphine or phosphine oxide.
Substitution: The pyridine ring can undergo electrophilic or nucleophilic substitution reactions, leading to the formation of various derivatives.
Common Reagents and Conditions
Oxidation: Common oxidizing agents include hydrogen peroxide, potassium permanganate, and chromium trioxide.
Reduction: Reducing agents such as lithium aluminum hydride or sodium borohydride are used.
Substitution: Reagents like alkyl halides, acyl chlorides, and sulfonyl chlorides are employed under appropriate conditions.
Major Products Formed
The major products formed from these reactions include phosphonic acids, phosphonates, phosphines, and various substituted pyridine derivatives. These products have diverse applications in organic synthesis and material science.
Scientific Research Applications
3-(Diethoxyphosphorylmethyl)pyridine has a wide range of applications in scientific research, including:
Chemistry: It is used as a building block in the synthesis of more complex organic molecules
Biology: The compound is studied for its potential biological activities, including antimicrobial and anticancer properties. It serves as a precursor for the synthesis of bioactive molecules.
Medicine: Research is ongoing to explore its potential as a pharmaceutical intermediate. Its derivatives may exhibit therapeutic effects in various diseases.
Industry: In industrial applications, it is used in the production of specialty chemicals, agrochemicals, and materials with specific properties.
Mechanism of Action
The mechanism of action of 3-(Diethoxyphosphorylmethyl)pyridine involves its interaction with molecular targets through its phosphoryl group. This group can form strong bonds with metal ions, enzymes, and other biomolecules, influencing their activity. The compound may inhibit or activate specific pathways, depending on the nature of the target. Detailed studies are required to elucidate the exact molecular mechanisms and pathways involved.
Comparison with Similar Compounds
Similar Compounds
3-(Diethoxyphosphorylmethyl)furan: A similar compound where the pyridine ring is replaced with a furan ring.
3-(Diethoxyphosphorylmethyl)thiophene: A thiophene analog with similar functional groups.
3-(Diethoxyphosphorylmethyl)benzene: A benzene derivative with the same phosphoryl group.
Uniqueness
3-(Diethoxyphosphorylmethyl)pyridine is unique due to the presence of the pyridine ring, which imparts distinct electronic and steric properties. This makes it more versatile in certain chemical reactions compared to its analogs. The nitrogen atom in the pyridine ring can participate in coordination chemistry, enhancing its utility in various applications.
Properties
IUPAC Name |
3-(diethoxyphosphorylmethyl)pyridine | |
---|---|---|
Details | Computed by LexiChem 2.6.6 (PubChem release 2019.06.18) | |
Source | PubChem | |
URL | https://pubchem.ncbi.nlm.nih.gov | |
Description | Data deposited in or computed by PubChem | |
InChI |
InChI=1S/C10H16NO3P/c1-3-13-15(12,14-4-2)9-10-6-5-7-11-8-10/h5-8H,3-4,9H2,1-2H3 | |
Details | Computed by InChI 1.0.5 (PubChem release 2019.06.18) | |
Source | PubChem | |
URL | https://pubchem.ncbi.nlm.nih.gov | |
Description | Data deposited in or computed by PubChem | |
InChI Key |
GSYCFXHFGKXCLR-UHFFFAOYSA-N | |
Details | Computed by InChI 1.0.5 (PubChem release 2019.06.18) | |
Source | PubChem | |
URL | https://pubchem.ncbi.nlm.nih.gov | |
Description | Data deposited in or computed by PubChem | |
Canonical SMILES |
CCOP(=O)(CC1=CN=CC=C1)OCC | |
Details | Computed by OEChem 2.1.5 (PubChem release 2019.06.18) | |
Source | PubChem | |
URL | https://pubchem.ncbi.nlm.nih.gov | |
Description | Data deposited in or computed by PubChem | |
Molecular Formula |
C10H16NO3P | |
Details | Computed by PubChem 2.1 (PubChem release 2019.06.18) | |
Source | PubChem | |
URL | https://pubchem.ncbi.nlm.nih.gov | |
Description | Data deposited in or computed by PubChem | |
Molecular Weight |
229.21 g/mol | |
Details | Computed by PubChem 2.1 (PubChem release 2021.05.07) | |
Source | PubChem | |
URL | https://pubchem.ncbi.nlm.nih.gov | |
Description | Data deposited in or computed by PubChem | |
Synthesis routes and methods
Procedure details
Retrosynthesis Analysis
AI-Powered Synthesis Planning: Our tool employs the Template_relevance Pistachio, Template_relevance Bkms_metabolic, Template_relevance Pistachio_ringbreaker, Template_relevance Reaxys, Template_relevance Reaxys_biocatalysis model, leveraging a vast database of chemical reactions to predict feasible synthetic routes.
One-Step Synthesis Focus: Specifically designed for one-step synthesis, it provides concise and direct routes for your target compounds, streamlining the synthesis process.
Accurate Predictions: Utilizing the extensive PISTACHIO, BKMS_METABOLIC, PISTACHIO_RINGBREAKER, REAXYS, REAXYS_BIOCATALYSIS database, our tool offers high-accuracy predictions, reflecting the latest in chemical research and data.
Strategy Settings
Precursor scoring | Relevance Heuristic |
---|---|
Min. plausibility | 0.01 |
Model | Template_relevance |
Template Set | Pistachio/Bkms_metabolic/Pistachio_ringbreaker/Reaxys/Reaxys_biocatalysis |
Top-N result to add to graph | 6 |
Feasible Synthetic Routes
Disclaimer and Information on In-Vitro Research Products
Please be aware that all articles and product information presented on BenchChem are intended solely for informational purposes. The products available for purchase on BenchChem are specifically designed for in-vitro studies, which are conducted outside of living organisms. In-vitro studies, derived from the Latin term "in glass," involve experiments performed in controlled laboratory settings using cells or tissues. It is important to note that these products are not categorized as medicines or drugs, and they have not received approval from the FDA for the prevention, treatment, or cure of any medical condition, ailment, or disease. We must emphasize that any form of bodily introduction of these products into humans or animals is strictly prohibited by law. It is essential to adhere to these guidelines to ensure compliance with legal and ethical standards in research and experimentation.