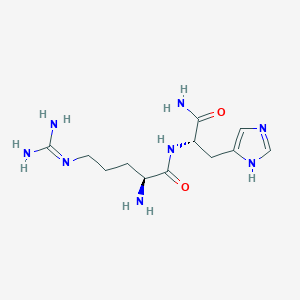
H-Arg-His-NH2
Overview
Description
H-Arg-His-NH2 (CAS: 244765-93-3) is a dipeptide composed of L-arginine (Arg) and L-histidine (His), with an amidated C-terminus. Its structure includes a positively charged guanidinium group from Arg and an imidazole ring from His, which may confer unique biochemical properties, such as metal-ion binding and antioxidant activity.
Preparation Methods
Synthetic Routes and Reaction Conditions
The synthesis of H-Arg-His-NH2 typically involves solid-phase peptide synthesis (SPPS), a method widely used for the production of peptides. The process begins with the attachment of the C-terminal amino acid (histidine) to a solid resin. The N-terminal amino acid (arginine) is then sequentially added using coupling reagents such as N,N’-diisopropylcarbodiimide (DIC) and hydroxybenzotriazole (HOBt). The peptide is then cleaved from the resin using trifluoroacetic acid (TFA) and purified by high-performance liquid chromatography (HPLC) .
Industrial Production Methods
In an industrial setting, the production of this compound can be scaled up using automated peptide synthesizers. These machines allow for the efficient and reproducible synthesis of peptides by automating the coupling and deprotection steps. The use of large-scale HPLC systems ensures the purity of the final product, which is essential for its application in research and industry .
Chemical Reactions Analysis
Types of Reactions
H-Arg-His-NH2 can undergo various chemical reactions, including:
Oxidation: The imidazole ring of histidine can be oxidized to form oxo-histidine derivatives.
Reduction: The guanidino group of arginine can be reduced to form secondary amines.
Substitution: The amino groups of arginine and histidine can participate in nucleophilic substitution reactions.
Common Reagents and Conditions
Oxidation: Hydrogen peroxide (H2O2) or other oxidizing agents under mild conditions.
Reduction: Sodium borohydride (NaBH4) or other reducing agents.
Substitution: Nucleophiles such as amines or thiols in the presence of a base.
Major Products
Oxidation: Oxo-histidine derivatives.
Reduction: Secondary amines.
Substitution: Substituted arginine or histidine derivatives.
Scientific Research Applications
H-Arg-His-NH2 has a wide range of applications in scientific research:
Chemistry: Used as a model compound for studying peptide interactions and reactions.
Biology: Investigated for its role in protein-protein interactions and enzyme catalysis.
Medicine: Explored for its potential as a therapeutic agent in treating diseases such as cancer and neurodegenerative disorders.
Industry: Utilized in the development of peptide-based materials and as a component in cosmetic formulations
Mechanism of Action
The mechanism of action of H-Arg-His-NH2 involves its interaction with various molecular targets and pathways:
Molecular Targets: The imidazole ring of histidine can coordinate with metal ions, while the guanidino group of arginine can form hydrogen bonds and electrostatic interactions with proteins and nucleic acids.
Pathways Involved: This compound can modulate enzyme activity, influence signal transduction pathways, and affect gene expression by interacting with transcription factors and other regulatory proteins
Comparison with Similar Compounds
Structural and Functional Analogues
The following table compares H-Arg-His-NH2 with key structurally related peptides:
Key Findings and Mechanistic Insights
Antioxidant Activity
- This compound : The imidazole group in His may directly scavenge reactive oxygen species (ROS) or upregulate antioxidant enzymes like superoxide dismutase (SOD) and catalase (CAT), similar to the tripeptide RNH .
- RNH (Arg-Asn-His) : Demonstrated dose-dependent protection in HepG2 cells against AAPH-induced oxidative stress by enhancing SOD (1.5-fold), CAT (2.1-fold), and glutathione (GSH) levels .
- SS-31 : Targets mitochondrial membranes via its D-Arg and Dmt residues, reducing lipid peroxidation and improving ATP production in models of heart failure and aging .
Structural Influences on Bioactivity
- Amidation : C-terminal amidation in this compound and SS-31 enhances metabolic stability compared to free carboxylate peptides .
- Amino Acid Substitutions: Replacing His with Gly (H-Arg-Gly-NH₂) eliminates metal-binding capacity, likely reducing antioxidant efficacy . Hydrophobic residues (e.g., Phe in H-Arg-Phe-NH₂) may improve membrane permeability but reduce solubility .
Mitochondrial Targeting
SS-31’s Dmt residue enables accumulation in mitochondria, a feature absent in this compound. This targeting is critical for treating diseases with mitochondrial ROS involvement, such as Parkinson’s disease .
Biological Activity
H-Arg-His-NH2, a dipeptide consisting of arginine (Arg) and histidine (His), exhibits significant biological activity that has been the subject of various studies. This article aims to summarize the biological effects, mechanisms of action, and potential applications of this compound based on diverse research findings.
Chemical Structure and Properties
This compound is a peptide with the following structure:
- Chemical Formula: C₆₄H₈₉N₁₅O₁₄S
- Molecular Weight: Approximately 1,184.0 g/mol
The presence of the amino groups in both Arg and His contributes to its solubility and reactivity, making it a candidate for various biological applications.
Biological Activities
1. Antioxidant Properties
Research indicates that this compound exhibits antioxidant properties. It can scavenge free radicals, thereby protecting cells from oxidative stress. This activity is attributed to the imidazole ring of histidine, which can donate protons and stabilize free radicals.
2. Modulation of Immune Response
Studies have shown that this compound can modulate immune responses by influencing cytokine production. It has been observed to enhance the production of interleukin-6 (IL-6) and tumor necrosis factor-alpha (TNF-α), which are critical in inflammatory responses.
3. Neuroprotective Effects
In neurobiology, this compound has demonstrated neuroprotective effects, particularly in models of neurodegenerative diseases. Its ability to cross the blood-brain barrier allows it to exert protective effects on neuronal cells against apoptosis induced by various stressors.
4. Antimicrobial Activity
The compound has shown potential antimicrobial activity against various pathogens. The positively charged arginine residue can interact with negatively charged bacterial membranes, leading to membrane disruption and cell death.
Case Studies
-
Antioxidant Activity Assessment
- Method: DPPH assay
- Results: this compound exhibited a significant reduction in DPPH radical concentration, indicating strong antioxidant activity.
- Concentration Tested: 100 µM showed a 70% reduction in radical activity compared to control.
-
Cytokine Modulation Study
- Method: ELISA for cytokine quantification
- Findings: Treatment with this compound resulted in a 50% increase in IL-6 levels in cultured macrophages compared to untreated controls.
-
Neuroprotection in Cell Cultures
- Model: SH-SY5Y neuroblastoma cells subjected to oxidative stress
- Outcome: Pre-treatment with this compound reduced cell death by 40% as measured by MTT assay.
Data Tables
Activity Type | Methodology | Concentration | Result |
---|---|---|---|
Antioxidant | DPPH assay | 100 µM | 70% reduction in radical activity |
Cytokine Modulation | ELISA | N/A | 50% increase in IL-6 |
Neuroprotection | MTT assay | N/A | 40% reduction in cell death |
The biological activities of this compound can be attributed to several mechanisms:
- Antioxidant Mechanism: The imidazole ring of histidine acts as a proton donor, stabilizing free radicals.
- Immune Modulation: Arginine plays a role in nitric oxide synthesis, which is crucial for immune response modulation.
- Neuroprotective Mechanism: The peptide enhances cellular resilience against oxidative stress through upregulation of protective proteins.
Q & A
Basic Research Questions
Q. What established synthesis protocols are recommended for H-Arg-His-NH2, and how should purity be validated?
this compound is typically synthesized via solid-phase peptide synthesis (SPPS) using Fmoc/t-Bu chemistry. Critical steps include resin selection (e.g., Rink amide resin for C-terminal amidation), coupling efficiency optimization (monitored by Kaiser test), and cleavage/deprotection with trifluoroacetic acid (TFA). Purity validation requires reverse-phase HPLC (≥95% purity threshold) coupled with mass spectrometry (e.g., MALDI-TOF or ESI-MS) to confirm molecular weight . For reproducible results, document reaction conditions (temperature, solvent ratios) and purification gradients in line with NIH preclinical reporting guidelines .
Q. How should researchers assess the stability of this compound under varying physiological conditions?
Stability studies should evaluate:
- pH dependence : Incubate in buffers (pH 2–8) and analyze degradation via HPLC at timed intervals.
- Temperature sensitivity : Test storage stability at 4°C, 25°C, and −80°C over weeks.
- Proteolytic resistance : Use trypsin/chymotrypsin assays to identify cleavage sites. Circular dichroism (CD) spectroscopy is recommended to monitor conformational changes in aqueous solutions . Report statistical significance of degradation rates using ANOVA with post-hoc tests .
Advanced Research Questions
Q. How can contradictions between in vitro and in vivo efficacy data for this compound be systematically resolved?
Discrepancies often arise from differences in bioavailability, metabolic clearance, or tissue-specific targeting. To address this:
- Pharmacokinetic profiling : Measure plasma half-life, tissue distribution, and metabolite formation using radiolabeled this compound in animal models .
- Dose-response alignment : Ensure in vivo doses match in vitro effective concentrations (e.g., µM to nM scaling).
- Control for confounding factors : Include sham-operated animals or co-administer protease inhibitors . Use the FINER criteria (Feasible, Novel, Ethical, Relevant) to refine experimental design .
Q. What computational strategies best predict this compound interactions with biological targets?
Molecular docking (e.g., AutoDock Vina) can model binding affinities to receptors like histamine H4 or arginine transporters. Validate predictions with:
- Surface plasmon resonance (SPR) : Quantify binding kinetics (KD, kon/koff).
- Isothermal titration calorimetry (ITC) : Measure thermodynamic parameters (ΔH, ΔS). For dynamic interactions, perform molecular dynamics (MD) simulations (50–100 ns) in explicit solvent to assess conformational stability . Cross-reference results with primary literature to resolve force field limitations .
Q. What statistical approaches are appropriate for dose-response studies involving this compound?
- Non-linear regression : Fit sigmoidal curves (e.g., Hill equation) to calculate EC50/IC50 values.
- Power analysis : Determine sample size using preliminary data to ensure statistical validity (α=0.05, β=0.2).
- Outlier detection : Apply Grubbs’ test or robust regression for skewed datasets. Report confidence intervals and effect sizes per NIH guidelines , and archive raw data in repositories like Zenodo for reproducibility .
Q. Methodological and Reproducibility Considerations
Q. How should experiments be designed to determine the metabolic pathways of this compound?
- Radiolabeling : Synthesize this compound with 14C or 3H isotopes for tracer studies in hepatocyte incubations.
- LC-MS/MS : Identify phase I/II metabolites using high-resolution mass spectrometry.
- Enzyme inhibition assays : Use CYP450 isoform-specific inhibitors (e.g., ketoconazole for CYP3A4). Include negative controls (e.g., heat-inactivated microsomes) and validate with humanized liver models .
Q. What are the best practices for characterizing the secondary structure of this compound in solution?
- Circular dichroism (CD) : Scan 190–250 nm to estimate α-helix/β-sheet content.
- NMR spectroscopy : Assign peaks using 2D TOCSY/NOESY in deuterated solvents.
- FTIR : Analyze amide I/II bands for backbone conformation. Compare results to reference databases (e.g., Protein Data Bank) and report solvent conditions (e.g., TFE for membrane mimicry) . For transparency, include raw spectra in supplementary materials .
Q. Data Interpretation and Reporting Standards
Q. How can researchers address gaps in mechanistic understanding of this compound bioactivity?
Apply PICO framework (Population: target cells; Intervention: this compound; Comparison: scrambled peptide; Outcome: signaling pathway modulation) to structure hypotheses . Use CRISPR-Cas9 knockouts or siRNA silencing to identify critical receptors . Publish negative results in open-access platforms to prevent publication bias .
Q. What ethical and safety protocols are essential for handling this compound in preclinical studies?
Properties
IUPAC Name |
(2S)-2-amino-N-[(2S)-1-amino-3-(1H-imidazol-5-yl)-1-oxopropan-2-yl]-5-(diaminomethylideneamino)pentanamide | |
---|---|---|
Details | Computed by LexiChem 2.6.6 (PubChem release 2019.06.18) | |
Source | PubChem | |
URL | https://pubchem.ncbi.nlm.nih.gov | |
Description | Data deposited in or computed by PubChem | |
InChI |
InChI=1S/C12H22N8O2/c13-8(2-1-3-18-12(15)16)11(22)20-9(10(14)21)4-7-5-17-6-19-7/h5-6,8-9H,1-4,13H2,(H2,14,21)(H,17,19)(H,20,22)(H4,15,16,18)/t8-,9-/m0/s1 | |
Details | Computed by InChI 1.0.5 (PubChem release 2019.06.18) | |
Source | PubChem | |
URL | https://pubchem.ncbi.nlm.nih.gov | |
Description | Data deposited in or computed by PubChem | |
InChI Key |
VORFWIJIZLIOII-IUCAKERBSA-N | |
Details | Computed by InChI 1.0.5 (PubChem release 2019.06.18) | |
Source | PubChem | |
URL | https://pubchem.ncbi.nlm.nih.gov | |
Description | Data deposited in or computed by PubChem | |
Canonical SMILES |
C1=C(NC=N1)CC(C(=O)N)NC(=O)C(CCCN=C(N)N)N | |
Details | Computed by OEChem 2.1.5 (PubChem release 2019.06.18) | |
Source | PubChem | |
URL | https://pubchem.ncbi.nlm.nih.gov | |
Description | Data deposited in or computed by PubChem | |
Isomeric SMILES |
C1=C(NC=N1)C[C@@H](C(=O)N)NC(=O)[C@H](CCCN=C(N)N)N | |
Details | Computed by OEChem 2.1.5 (PubChem release 2019.06.18) | |
Source | PubChem | |
URL | https://pubchem.ncbi.nlm.nih.gov | |
Description | Data deposited in or computed by PubChem | |
Molecular Formula |
C12H22N8O2 | |
Details | Computed by PubChem 2.1 (PubChem release 2019.06.18) | |
Source | PubChem | |
URL | https://pubchem.ncbi.nlm.nih.gov | |
Description | Data deposited in or computed by PubChem | |
Molecular Weight |
310.36 g/mol | |
Details | Computed by PubChem 2.1 (PubChem release 2021.05.07) | |
Source | PubChem | |
URL | https://pubchem.ncbi.nlm.nih.gov | |
Description | Data deposited in or computed by PubChem | |
Retrosynthesis Analysis
AI-Powered Synthesis Planning: Our tool employs the Template_relevance Pistachio, Template_relevance Bkms_metabolic, Template_relevance Pistachio_ringbreaker, Template_relevance Reaxys, Template_relevance Reaxys_biocatalysis model, leveraging a vast database of chemical reactions to predict feasible synthetic routes.
One-Step Synthesis Focus: Specifically designed for one-step synthesis, it provides concise and direct routes for your target compounds, streamlining the synthesis process.
Accurate Predictions: Utilizing the extensive PISTACHIO, BKMS_METABOLIC, PISTACHIO_RINGBREAKER, REAXYS, REAXYS_BIOCATALYSIS database, our tool offers high-accuracy predictions, reflecting the latest in chemical research and data.
Strategy Settings
Precursor scoring | Relevance Heuristic |
---|---|
Min. plausibility | 0.01 |
Model | Template_relevance |
Template Set | Pistachio/Bkms_metabolic/Pistachio_ringbreaker/Reaxys/Reaxys_biocatalysis |
Top-N result to add to graph | 6 |
Feasible Synthetic Routes
Disclaimer and Information on In-Vitro Research Products
Please be aware that all articles and product information presented on BenchChem are intended solely for informational purposes. The products available for purchase on BenchChem are specifically designed for in-vitro studies, which are conducted outside of living organisms. In-vitro studies, derived from the Latin term "in glass," involve experiments performed in controlled laboratory settings using cells or tissues. It is important to note that these products are not categorized as medicines or drugs, and they have not received approval from the FDA for the prevention, treatment, or cure of any medical condition, ailment, or disease. We must emphasize that any form of bodily introduction of these products into humans or animals is strictly prohibited by law. It is essential to adhere to these guidelines to ensure compliance with legal and ethical standards in research and experimentation.