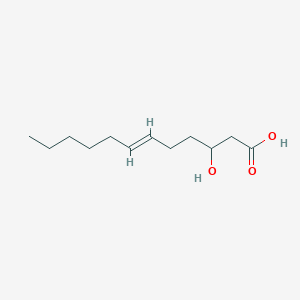
3-Hydroxy-6-dodecenoic acid
Overview
Description
3-Hydroxy-6-dodecenoic acid is an organic compound with the molecular formula C12H22O3. It is a hydroxy fatty acid characterized by the presence of a hydroxyl group at the third carbon and a double bond between the sixth and seventh carbons in the dodecenoic acid chain.
Mechanism of Action
Target of Action
3-Hydroxy-6-dodecenoic acid is primarily targeted against fungal species , particularly those belonging to the Aspergillus genus . These fungi are known to cause food spoilage and produce harmful mycotoxins .
Mode of Action
The compound is produced by certain strains of Lactobacillus, a type of lactic acid bacteria . It acts as an antifungal metabolite , inhibiting the growth of fungi .
Biochemical Pathways
This compound is generated from 3-hydroxy-fatty acyl ACPs through a process involving desaturase and thioesterase action . This compound is part of a larger group of metabolites that contribute to the antifungal potential of Lactobacillus .
Pharmacokinetics
Given its role as a natural food preservative, it is likely that the compound exhibits good stability and bioavailability .
Result of Action
The primary result of the action of this compound is the inhibition of fungal growth . This leads to a decrease in food spoilage and a reduction in the presence of harmful mycotoxins . The compound’s antimicrobial efficacy results from cell aggregation due to severe damage to the surface of vegetative cells and is enhanced in the presence of lactic and acetic acids .
Action Environment
The action of this compound is influenced by various environmental factors. For instance, its production by Lactobacillus is likely influenced by the nutrient availability in the environment . Furthermore, the compound’s stability and efficacy as a food preservative suggest that it is resistant to changes in temperature and pH, common environmental factors in food storage .
Biochemical Analysis
Biochemical Properties
3-Hydroxy-6-dodecenoic acid interacts with various enzymes, proteins, and other biomolecules in biochemical reactions . It is a medium-chain fatty acid, which means it has a moderate length and behaves similarly to other fatty acids in chemical terms . It is a solid at room temperature and has a characteristic odor of fatty acids .
Cellular Effects
It has been found to have antifungal activity, especially against Aspergillus species . This suggests that it may influence cell function, including impacts on cell signaling pathways, gene expression, and cellular metabolism .
Molecular Mechanism
It is known to exert its effects at the molecular level, potentially through binding interactions with biomolecules, enzyme inhibition or activation, and changes in gene expression .
Metabolic Pathways
This compound is involved in various metabolic pathways. It interacts with enzymes and cofactors, and may also affect metabolic flux or metabolite levels
Preparation Methods
Synthetic Routes and Reaction Conditions: 3-Hydroxy-6-dodecenoic acid can be synthesized through several methods. One common approach involves the Doebner condensation of aldehydes with malonic acid, followed by dehydration . Another method includes the Reformatsky reaction of aldehydes with ethyl bromoacetate, which is then followed by dehydration .
Industrial Production Methods: Industrial production of this compound typically involves the use of biotechnological processes. For instance, certain strains of Lactobacillus plantarum have been reported to produce this compound as a part of their metabolic processes .
Chemical Reactions Analysis
Types of Reactions: 3-Hydroxy-6-dodecenoic acid undergoes various chemical reactions, including:
Oxidation: The hydroxyl group can be oxidized to form a keto group.
Reduction: The double bond can be reduced to form 3-hydroxy-dodecanoic acid.
Substitution: The hydroxyl group can be substituted with other functional groups under appropriate conditions.
Common Reagents and Conditions:
Oxidation: Common oxidizing agents include potassium permanganate and chromium trioxide.
Reduction: Catalytic hydrogenation using palladium on carbon is a typical method.
Substitution: Reagents such as thionyl chloride can be used to substitute the hydroxyl group with a chlorine atom.
Major Products:
Oxidation: 3-Keto-6-dodecenoic acid.
Reduction: 3-Hydroxy-dodecanoic acid.
Substitution: 3-Chloro-6-dodecenoic acid.
Scientific Research Applications
3-Hydroxy-6-dodecenoic acid has a wide range of applications in scientific research:
Chemistry: It is used as a building block in the synthesis of more complex molecules.
Biology: The compound exhibits antifungal properties and is studied for its potential use in bio-preservation.
Industry: It is used in the production of biodegradable plastics and other environmentally friendly materials.
Comparison with Similar Compounds
- 3-Hydroxydecanoic acid
- 3-Hydroxy-5-cis-dodecenoic acid
- 3-Hydroxytetradecanoic acid
Comparison: 3-Hydroxy-6-dodecenoic acid is unique due to its specific structure, which includes a hydroxyl group at the third carbon and a double bond between the sixth and seventh carbons. This structure imparts distinct chemical and biological properties compared to other similar compounds. For instance, 3-Hydroxydecanoic acid lacks the double bond, which affects its reactivity and biological activity .
Properties
IUPAC Name |
(E)-3-hydroxydodec-6-enoic acid | |
---|---|---|
Details | Computed by LexiChem 2.6.6 (PubChem release 2019.06.18) | |
Source | PubChem | |
URL | https://pubchem.ncbi.nlm.nih.gov | |
Description | Data deposited in or computed by PubChem | |
InChI |
InChI=1S/C12H22O3/c1-2-3-4-5-6-7-8-9-11(13)10-12(14)15/h6-7,11,13H,2-5,8-10H2,1H3,(H,14,15)/b7-6+ | |
Details | Computed by InChI 1.0.5 (PubChem release 2019.06.18) | |
Source | PubChem | |
URL | https://pubchem.ncbi.nlm.nih.gov | |
Description | Data deposited in or computed by PubChem | |
InChI Key |
PCZWZQDPYMZGMC-VOTSOKGWSA-N | |
Details | Computed by InChI 1.0.5 (PubChem release 2019.06.18) | |
Source | PubChem | |
URL | https://pubchem.ncbi.nlm.nih.gov | |
Description | Data deposited in or computed by PubChem | |
Canonical SMILES |
CCCCCC=CCCC(CC(=O)O)O | |
Details | Computed by OEChem 2.1.5 (PubChem release 2019.06.18) | |
Source | PubChem | |
URL | https://pubchem.ncbi.nlm.nih.gov | |
Description | Data deposited in or computed by PubChem | |
Isomeric SMILES |
CCCCC/C=C/CCC(CC(=O)O)O | |
Details | Computed by OEChem 2.1.5 (PubChem release 2019.06.18) | |
Source | PubChem | |
URL | https://pubchem.ncbi.nlm.nih.gov | |
Description | Data deposited in or computed by PubChem | |
Molecular Formula |
C12H22O3 | |
Details | Computed by PubChem 2.1 (PubChem release 2019.06.18) | |
Source | PubChem | |
URL | https://pubchem.ncbi.nlm.nih.gov | |
Description | Data deposited in or computed by PubChem | |
Molecular Weight |
214.30 g/mol | |
Details | Computed by PubChem 2.1 (PubChem release 2021.05.07) | |
Source | PubChem | |
URL | https://pubchem.ncbi.nlm.nih.gov | |
Description | Data deposited in or computed by PubChem | |
Retrosynthesis Analysis
AI-Powered Synthesis Planning: Our tool employs the Template_relevance Pistachio, Template_relevance Bkms_metabolic, Template_relevance Pistachio_ringbreaker, Template_relevance Reaxys, Template_relevance Reaxys_biocatalysis model, leveraging a vast database of chemical reactions to predict feasible synthetic routes.
One-Step Synthesis Focus: Specifically designed for one-step synthesis, it provides concise and direct routes for your target compounds, streamlining the synthesis process.
Accurate Predictions: Utilizing the extensive PISTACHIO, BKMS_METABOLIC, PISTACHIO_RINGBREAKER, REAXYS, REAXYS_BIOCATALYSIS database, our tool offers high-accuracy predictions, reflecting the latest in chemical research and data.
Strategy Settings
Precursor scoring | Relevance Heuristic |
---|---|
Min. plausibility | 0.01 |
Model | Template_relevance |
Template Set | Pistachio/Bkms_metabolic/Pistachio_ringbreaker/Reaxys/Reaxys_biocatalysis |
Top-N result to add to graph | 6 |
Feasible Synthetic Routes
Disclaimer and Information on In-Vitro Research Products
Please be aware that all articles and product information presented on BenchChem are intended solely for informational purposes. The products available for purchase on BenchChem are specifically designed for in-vitro studies, which are conducted outside of living organisms. In-vitro studies, derived from the Latin term "in glass," involve experiments performed in controlled laboratory settings using cells or tissues. It is important to note that these products are not categorized as medicines or drugs, and they have not received approval from the FDA for the prevention, treatment, or cure of any medical condition, ailment, or disease. We must emphasize that any form of bodily introduction of these products into humans or animals is strictly prohibited by law. It is essential to adhere to these guidelines to ensure compliance with legal and ethical standards in research and experimentation.