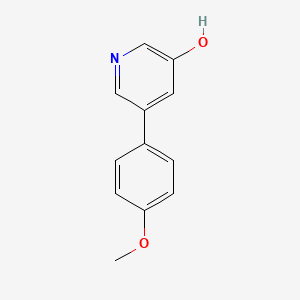
5-(4-Methoxyphenyl)pyridin-3-ol
Overview
Description
5-(4-Methoxyphenyl)pyridin-3-ol is a chemical compound with the empirical formula C6H7NO2 . It is a substituted pyridine with diverse functional groups and is an important structural motif found in numerous bioactive molecules .
Synthesis Analysis
The synthesis of 5-(4-Methoxyphenyl)pyridin-3-ol involves a ring cleavage methodology reaction. This method allows for the synthesis of 2-alkyl/aryl 3-electron-withdrawing groups (esters, sulfones, and phosphonates) 5-aminoaryl/phenol pyridines via the remodeling of 3-formyl (aza)indoles/benzofurans .Molecular Structure Analysis
The molecular structure of 5-(4-Methoxyphenyl)pyridin-3-ol is characterized by a pyridine ring, which is a simple six-membered heterocyclic scaffold. The biological activities and physical properties of pyridine analogs can be improved by introducing various functional groups into the pyridine scaffold .Chemical Reactions Analysis
The chemical reactions involved in the synthesis of 5-(4-Methoxyphenyl)pyridin-3-ol are based on the aldol-type reaction between 3-formyl (aza)indole and β-aminoacrylate .Physical And Chemical Properties Analysis
The physical and chemical properties of 5-(4-Methoxyphenyl)pyridin-3-ol can be influenced by the introduction of various functional groups into the pyridine scaffold .Scientific Research Applications
- Notably, 4-(2,6-Diphenyl-2H-pyrazolo [4,3-c]pyridin-7-yl)phenol exhibited low micromolar GI50 values and induced cell death pathways, including PARP-1 cleavage and caspase 9 activation .
- Its fluorescence properties enable both intensity-based and ratiometric pH sensing, making it valuable for biological and analytical applications .
- They selectively inhibit the linoleate oxygenase activity of ALOX15 , suggesting potential therapeutic applications .
- These structures have found use in drug discovery, enzyme inhibition, and other biomedical applications .
Antiproliferative Activity
Fluorescent pH Indicator
Linoleate Oxygenase Inhibition
Biological Targets
Photophysical Properties
Safety and Hazards
Future Directions
Mechanism of Action
Target of Action
5-(4-Methoxyphenyl)pyridin-3-ol, also known as Gaboxadol or THIP, primarily targets the GABA system . It interacts with the GABA receptors, particularly the α4β3δ GABA A receptors . These receptors play a crucial role in inhibitory neurotransmission in the central nervous system .
Mode of Action
Gaboxadol acts on the GABA system in a unique way, different from benzodiazepines and other sedatives . It is a supra-maximal agonist at α4β3δ GABA A receptors, a low-potency agonist at α1β3γ2, and a partial agonist at α4β3γ . Its affinity for this α4-containing subtype of the GABA A receptor is 10× greater than other non-α4 containing subtypes .
Biochemical Pathways
Gaboxadol’s action affects the GABAergic neurotransmission pathway . By acting as an agonist at specific GABA A receptors, it enhances the inhibitory effects of GABA in the central nervous system. This can lead to increased deep sleep, as reported by Lundbeck and Merck .
Result of Action
The primary result of Gaboxadol’s action is an increase in deep sleep . This is due to its unique interaction with the GABA system, enhancing inhibitory neurotransmission and promoting sleep. Development of gaboxadol was stopped due to concerns regarding safety and efficacy .
properties
IUPAC Name |
5-(4-methoxyphenyl)pyridin-3-ol | |
---|---|---|
Details | Computed by Lexichem TK 2.7.0 (PubChem release 2021.05.07) | |
Source | PubChem | |
URL | https://pubchem.ncbi.nlm.nih.gov | |
Description | Data deposited in or computed by PubChem | |
InChI |
InChI=1S/C12H11NO2/c1-15-12-4-2-9(3-5-12)10-6-11(14)8-13-7-10/h2-8,14H,1H3 | |
Details | Computed by InChI 1.0.6 (PubChem release 2021.05.07) | |
Source | PubChem | |
URL | https://pubchem.ncbi.nlm.nih.gov | |
Description | Data deposited in or computed by PubChem | |
InChI Key |
AFEIJQZBPSMOAE-UHFFFAOYSA-N | |
Details | Computed by InChI 1.0.6 (PubChem release 2021.05.07) | |
Source | PubChem | |
URL | https://pubchem.ncbi.nlm.nih.gov | |
Description | Data deposited in or computed by PubChem | |
Canonical SMILES |
COC1=CC=C(C=C1)C2=CC(=CN=C2)O | |
Details | Computed by OEChem 2.3.0 (PubChem release 2021.05.07) | |
Source | PubChem | |
URL | https://pubchem.ncbi.nlm.nih.gov | |
Description | Data deposited in or computed by PubChem | |
Molecular Formula |
C12H11NO2 | |
Details | Computed by PubChem 2.1 (PubChem release 2021.05.07) | |
Source | PubChem | |
URL | https://pubchem.ncbi.nlm.nih.gov | |
Description | Data deposited in or computed by PubChem | |
DSSTOX Substance ID |
DTXSID10682619 | |
Record name | 5-(4-Methoxyphenyl)pyridin-3-ol | |
Source | EPA DSSTox | |
URL | https://comptox.epa.gov/dashboard/DTXSID10682619 | |
Description | DSSTox provides a high quality public chemistry resource for supporting improved predictive toxicology. | |
Molecular Weight |
201.22 g/mol | |
Details | Computed by PubChem 2.1 (PubChem release 2021.05.07) | |
Source | PubChem | |
URL | https://pubchem.ncbi.nlm.nih.gov | |
Description | Data deposited in or computed by PubChem | |
Product Name |
5-(4-Methoxyphenyl)pyridin-3-ol | |
CAS RN |
1258609-27-6 | |
Record name | 5-(4-Methoxyphenyl)pyridin-3-ol | |
Source | EPA DSSTox | |
URL | https://comptox.epa.gov/dashboard/DTXSID10682619 | |
Description | DSSTox provides a high quality public chemistry resource for supporting improved predictive toxicology. | |
Retrosynthesis Analysis
AI-Powered Synthesis Planning: Our tool employs the Template_relevance Pistachio, Template_relevance Bkms_metabolic, Template_relevance Pistachio_ringbreaker, Template_relevance Reaxys, Template_relevance Reaxys_biocatalysis model, leveraging a vast database of chemical reactions to predict feasible synthetic routes.
One-Step Synthesis Focus: Specifically designed for one-step synthesis, it provides concise and direct routes for your target compounds, streamlining the synthesis process.
Accurate Predictions: Utilizing the extensive PISTACHIO, BKMS_METABOLIC, PISTACHIO_RINGBREAKER, REAXYS, REAXYS_BIOCATALYSIS database, our tool offers high-accuracy predictions, reflecting the latest in chemical research and data.
Strategy Settings
Precursor scoring | Relevance Heuristic |
---|---|
Min. plausibility | 0.01 |
Model | Template_relevance |
Template Set | Pistachio/Bkms_metabolic/Pistachio_ringbreaker/Reaxys/Reaxys_biocatalysis |
Top-N result to add to graph | 6 |
Feasible Synthetic Routes
Disclaimer and Information on In-Vitro Research Products
Please be aware that all articles and product information presented on BenchChem are intended solely for informational purposes. The products available for purchase on BenchChem are specifically designed for in-vitro studies, which are conducted outside of living organisms. In-vitro studies, derived from the Latin term "in glass," involve experiments performed in controlled laboratory settings using cells or tissues. It is important to note that these products are not categorized as medicines or drugs, and they have not received approval from the FDA for the prevention, treatment, or cure of any medical condition, ailment, or disease. We must emphasize that any form of bodily introduction of these products into humans or animals is strictly prohibited by law. It is essential to adhere to these guidelines to ensure compliance with legal and ethical standards in research and experimentation.