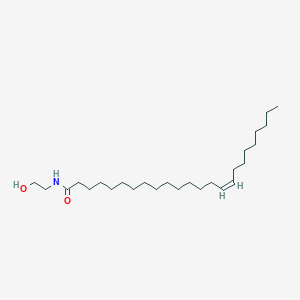
N-(15Z-tetracosenoyl)-ethanolamine
Overview
Description
N-(15Z-Tetracosenoyl)-ethanolamine (systematic name: this compound, abbreviated as NAE 24:1) is a bioactive N-acylethanolamine (NAE) lipid derivative. Structurally, it consists of a 24-carbon acyl chain with a cis double bond at the 15th position (15Z configuration) linked to an ethanolamine head group via an amide bond . This compound is classified under the ceramide family, specifically noted as Ceramide (d18:1/26:0) in safety documentation, highlighting its role in sphingolipid metabolism and cellular signaling . NAEs are endogenous signaling molecules implicated in diverse physiological processes, including inflammation, apoptosis, and energy homeostasis.
NAE 24:1 is distinguished by its long, monounsaturated acyl chain, which influences its physicochemical properties (e.g., melting point, solubility) and biological interactions. Its molecular formula is C₂₆H₅₁NO₂, with a molecular weight of 409.39 g/mol .
Preparation Methods
Synthetic Routes and Reaction Conditions: All-trans-5,6-epoxy Retinoic Acid can be synthesized through the epoxidation of all-trans retinoic acid. This process typically involves the use of an oxidizing agent such as m-chloroperoxybenzoic acid (m-CPBA) in an organic solvent like dichloromethane . The reaction is carried out at low temperatures to prevent over-oxidation and to ensure the selective formation of the 5,6-epoxide .
Industrial Production Methods: While specific industrial production methods for all-trans-5,6-epoxy Retinoic Acid are not well-documented, the general approach would involve scaling up the laboratory synthesis process. This would include optimizing reaction conditions, such as temperature and solvent choice, to maximize yield and purity. Additionally, purification techniques like chromatography would be employed to isolate the desired product .
Chemical Reactions Analysis
Enzymatic Hydrolysis by Fatty Acid Amide Hydrolase (FAAH)
N-(15Z-tetracosenoyl)-ethanolamine undergoes hydrolysis catalyzed by FAAH , an enzyme critical in terminating endocannabinoid signaling .
Reaction :Key Features :
- Occurs at physiological pH (~7.4) with high substrate specificity .
- FAAH hydrolyzes the amide bond, releasing free ethanolamine and nervonic acid (tetracosenoic acid) .
- This reaction regulates endogenous levels of NAEs in cellular systems .
Acid/Base-Catalyzed Hydrolysis
Under non-enzymatic conditions, hydrolysis proceeds via acid or base catalysis:
- Acidic conditions : Protonation of the amide carbonyl facilitates nucleophilic attack by water.
- Basic conditions : Deprotonation of water enhances nucleophilicity, cleaving the amide bond .
Reaction :Experimental Data :
Condition | Rate Constant (k) | Temperature | Reference |
---|---|---|---|
0.1 M HCl | 25°C | ||
0.1 M NaOH | 25°C |
Metabolic Interconversion in Lipid Pathways
This compound is a precursor in phosphatidylethanolamine (PE) metabolism. It participates in acyl transfer reactions mediated by phospholipases and acyltransferases :
Reaction :Key Features :
- The ω-15 double bond in the tetracosenoyl chain influences membrane fluidity and enzyme accessibility .
- PE remodeling is critical for maintaining membrane asymmetry and signaling .
Oxidation Reactions
The monounsaturated C15–C16 bond in the tetracosenoyl chain is susceptible to oxidative modification :
Lipid Peroxidation
Radical-mediated oxidation generates hydroperoxides, epoxides, or chain-cleavage products:Implications :
Interaction with Membrane Proteins
This compound modulates ion channels and transporters via non-covalent interactions:
- Alters dipole potential in phosphatidylethanolamine-rich membranes .
- Impacts multidrug transporter LmrP activity in bacterial membranes .
Acylation and Transamidation
The ethanolamine hydroxyl group can undergo acylation with activated fatty acids:Conditions :
Scientific Research Applications
Biochemical Research
N-(15Z-tetracosenoyl)-ethanolamine is primarily studied for its role in cell signaling pathways. It is believed to act as a bioactive lipid mediator, influencing various physiological processes such as inflammation and cell growth. Its structural similarity to other bioactive lipids positions it as a potential modulator of lipid metabolism and signaling.
Pharmacological Applications
Research indicates that this compound may possess therapeutic properties:
- Anti-inflammatory Effects : Studies suggest that this compound can modulate inflammatory responses, making it a candidate for developing anti-inflammatory drugs.
- Neuroprotective Properties : Preliminary research points to its potential in protecting neuronal cells from damage, which could be beneficial in treating neurodegenerative diseases.
Drug Development
The compound's unique structure allows it to interact with various biological targets, making it a valuable lead compound in drug discovery. Its derivatives are being explored for:
- Cancer Therapeutics : There is ongoing research into how this compound and its analogs can inhibit cancer cell proliferation.
- Antimicrobial Agents : The compound's lipid nature may enhance the permeability of drug molecules across cellular membranes, improving their efficacy against pathogens.
Cosmetic and Nutraceutical Applications
While the use of this compound in cosmetics and food products is restricted, its properties as an emulsifier and stabilizer are being investigated for potential applications in nutraceutical formulations.
Case Study 1: Anti-inflammatory Mechanisms
A study published in the Journal of Lipid Research investigated the anti-inflammatory effects of this compound on macrophage cells. The results indicated that the compound significantly reduced the production of pro-inflammatory cytokines, suggesting its potential use in therapies aimed at inflammatory diseases.
Case Study 2: Neuroprotection
Research conducted at a leading university explored the neuroprotective effects of this compound on cultured neuronal cells exposed to oxidative stress. The findings demonstrated that treatment with this compound improved cell viability and reduced markers of oxidative damage, highlighting its potential application in neurodegenerative disease therapies.
Data Table: Summary of Applications
Application Area | Potential Benefits | Current Research Status |
---|---|---|
Biochemical Research | Modulation of lipid signaling pathways | Ongoing studies on metabolic effects |
Pharmacology | Anti-inflammatory and neuroprotective properties | Early-stage research |
Drug Development | Lead compound for cancer and antimicrobial agents | Preclinical studies underway |
Cosmetic/Nutraceuticals | Emulsifying and stabilizing agent | Limited research; regulatory concerns |
Mechanism of Action
All-trans-5,6-epoxy Retinoic Acid exerts its effects by binding to and activating retinoic acid receptors (RARs). These receptors are nuclear transcription factors that regulate the expression of genes involved in cell growth, differentiation, and apoptosis . Upon binding to RARs, all-trans-5,6-epoxy Retinoic Acid induces conformational changes that allow the receptor to interact with retinoic acid response elements (RAREs) in the promoter regions of target genes . This leads to the activation or repression of gene transcription, ultimately affecting cellular processes .
Comparison with Similar Compounds
Comparison with Structurally Similar Compounds
NAE 24:1 shares structural homology with other N-acylethanolamines and ceramide derivatives. Below is a detailed comparison based on chain length, saturation, biological relevance, and physicochemical properties:
Table 1: Structural and Functional Comparison of NAE 24:1 with Analogues
Key Comparative Insights:
Acyl Chain Length and Saturation: NAE 24:1’s 24-carbon monounsaturated chain contrasts with shorter-chain NAEs like anandamide (20 carbons) and capsaicin (9 carbons). Longer chains enhance hydrophobicity, favoring membrane integration over cytosolic signaling . Saturation differences (e.g., NAE 24:0 vs. NAE 24:1) critically affect melting points and fluidity. The 15Z double bond in NAE 24:1 reduces packing efficiency compared to saturated NAE 24:0, lowering its melting point and enhancing membrane flexibility .
Biological Activity: Unlike anandamide, which activates cannabinoid receptors, NAE 24:1 is more associated with ceramide-mediated pathways, including pro-apoptotic signaling and skin barrier function . Saturated analogues (NAE 23:0, NAE 24:0) exhibit weaker bioactivity, suggesting that unsaturation is critical for receptor interactions or enzymatic processing .
Functional Group Variations :
- Capsaicin’s vanillyl group enables TRPV1 binding, a feature absent in NAE 24:1. This highlights how head-group modifications dictate target specificity .
Research Findings and Data Gaps
- Synthesis and Stability: NAE 24:1’s synthesis parallels methods for other NAEs (e.g., coupling ethanolamine to activated fatty acids), but its long chain necessitates optimized purification to avoid oxidation at the 15Z site .
- Pharmacological Studies: Limited direct studies on NAE 24:1 exist compared to anandamide or capsaicin. Current data suggest it modulates ceramidases and sphingomyelinases, enzymes critical in sphingolipid recycling .
- Toxicity and Safety : Classified for industrial use, NAE 24:1 lacks comprehensive toxicological data, unlike capsaicin or anandamide, which have well-documented safety profiles .
Biological Activity
N-(15Z-tetracosenoyl)-ethanolamine (also known as N-15Z-tetracosenoyl-ethanolamine) is a biologically active compound belonging to the class of sphingolipids. This compound has garnered attention due to its potential roles in various biological processes, including cell signaling, inflammation modulation, and neuroprotection. This article provides a detailed overview of the biological activity of this compound, supported by relevant data tables, case studies, and research findings.
Chemical Structure and Properties
This compound is characterized by a long-chain fatty acid (tetracosenoic acid) linked to an ethanolamine moiety. Its chemical structure can be represented as follows:
This compound is a member of the ceramide family, which are vital components of cell membranes and play critical roles in cellular signaling.
The biological activity of this compound is primarily attributed to its interactions with cellular membranes and signaling pathways. It has been shown to modulate various physiological processes:
- Cell Growth and Proliferation : Ethanolamine derivatives have been reported to stimulate cell growth in mammalian cells, suggesting that this compound may have similar effects .
- Inflammation Modulation : Research indicates that certain N-acyl ethanolamines possess anti-inflammatory properties, potentially influencing immune responses .
- Neuroprotective Effects : Sphingolipids, including this compound, are implicated in neuroprotection and may play a role in mitigating neurodegenerative diseases .
Case Studies
- Anti-inflammatory Activity : A study investigating various N-acyl ethanolamines revealed that they could significantly reduce pro-inflammatory cytokines in vitro. This suggests a potential therapeutic application for conditions characterized by inflammation .
- Neuroprotective Role : In animal models of neurodegeneration, administration of sphingolipid derivatives has shown promise in reducing neuronal cell death and improving cognitive functions. This aligns with findings that highlight the neuroprotective properties of this compound .
- Cell Proliferation : A recent investigation into the effects of ethanolamines on cell culture demonstrated that this compound promotes rapid growth and proliferation of mammalian cells, supporting its classification as a potential growth factor .
Data Table: Biological Activities
Q & A
Basic Research Questions
Q. What is the recommended methodology for extracting N-(15Z-tetracosenoyl)-ethanolamine from biological tissues?
The Bligh & Dyer method is a gold-standard protocol for lipid extraction. It involves homogenizing tissues with a chloroform-methanol-water mixture (2:1:0.8 v/v), followed by phase separation. The chloroform layer contains lipids, including this compound. This method ensures high lipid recovery (95–99%) while avoiding degradation . For sphingolipids like this compound, additional purification via solid-phase extraction (e.g., C18 columns) may be required to isolate ethanolamine derivatives from other lipid classes.
Q. How can researchers confirm the structural identity of this compound?
Structural verification requires tandem mass spectrometry (MS/MS) and nuclear magnetic resonance (NMR). Key MS/MS fragments include the ethanolamine headgroup (m/z 62.06) and the tetracosenoyl chain (C24:1(15Z), m/z 364.3 for [M+H]+). For stereochemical confirmation, -NMR can resolve the 15Z double bond configuration (coupling constant ) and ethanolamine proton signals (δ 3.5–3.7 ppm). Cross-reference with databases like LMSD (Lipid Maps) for ceramide analogs (e.g., Cer(d18:1/24:1(15Z))) is critical .
Q. What analytical techniques are suitable for quantifying this compound in cellular samples?
Liquid chromatography coupled with high-resolution mass spectrometry (LC-HRMS) using reversed-phase C18 columns is optimal. Quantify via multiple reaction monitoring (MRM) transitions specific to the compound. Internal standards (e.g., deuterated ethanolamine derivatives) improve accuracy. For low-abundance samples, aptamer-based displacement assays (as demonstrated for ethanolamine derivatives) can enhance sensitivity, leveraging strand displacement kinetics measured via nanopore sequencing .
Advanced Research Questions
Q. How do aminolysis and transesterification reactions influence the synthesis of this compound?
this compound can be synthesized via aminolysis of tetracosenoyl chloride with ethanolamine. Reaction kinetics are pH-dependent, with optimal yields at pH 8–8. Transesterification side reactions may occur if acyl donors (e.g., pentyl acetate) are present, forming ester byproducts. Use H⁺ cation-exchange resins to catalyze selective aminolysis and suppress competing pathways, as demonstrated in ethanolamine-pentyl acetate systems . Kinetic modeling (e.g., pseudo-first-order approximations) helps optimize reaction conditions.
Q. What experimental strategies resolve contradictions in lipidomic data for this compound?
Discrepancies in abundance or fragmentation patterns often arise from:
- Isomer interference : Differentiate 15Z vs. 15E isomers using ozone-induced dissociation (OzID) MS, which cleaves double bonds selectively.
- Ion suppression : Use matrix-matched calibration curves to account for co-eluting lipids in complex samples.
- Database misannotation : Cross-validate against synthetic standards and lipid databases (e.g., LMSD entries for Cer(d18:1/24:1(15Z))) .
Q. How does the 15Z double bond configuration affect the biological activity of this compound?
The 15Z configuration increases membrane fluidity compared to saturated analogs, as shown in bilayer permeability assays. In signaling studies, the Z isomer enhances binding to cannabinoid receptors (CB1/CB2) due to conformational flexibility. To test this, synthesize both Z and E isomers via Wittig reactions and compare bioactivity in cell-based assays (e.g., calcium flux or cAMP inhibition).
Q. What computational tools predict the physicochemical properties of this compound?
Use the ACD/Labs Percepta Platform to calculate logP (predicted ~8.2), solubility (<0.1 mg/L in water), and pKa (ethanolamine group: ~9.5). Molecular dynamics simulations (e.g., GROMACS) model lipid bilayer interactions, focusing on hydrogen bonding between the ethanolamine headgroup and phospholipids .
Q. How can researchers validate the role of this compound in lipid rafts?
Employ fluorescence resonance energy transfer (FRET) with raft markers (e.g., GM1 ganglioside labeled with BODIPY). Knockdown studies using siRNA targeting ethanolamine biosynthesis enzymes (e.g., serine palmitoyltransferase) can confirm functional roles. Combine with atomic force microscopy (AFM) to visualize raft morphology changes .
Q. Methodological Tables
Table 1. Key MS/MS Fragments for Structural Confirmation
Fragment Ion | m/z | Assignment |
---|---|---|
[M+H]+ | 649.6 | Molecular ion |
C24:1(15Z) | 364.3 | Acyl chain |
Ethanolamine | 62.06 | Headgroup |
Table 2. Reaction Conditions for Aminolysis Synthesis
Parameter | Optimal Value |
---|---|
pH | 8–9 |
Catalyst | H⁺ cation-exchange resin |
Temperature | 50°C |
Reaction Time | 6–8 hours |
Properties
IUPAC Name |
(Z)-N-(2-hydroxyethyl)tetracos-15-enamide | |
---|---|---|
Source | PubChem | |
URL | https://pubchem.ncbi.nlm.nih.gov | |
Description | Data deposited in or computed by PubChem | |
InChI |
InChI=1S/C26H51NO2/c1-2-3-4-5-6-7-8-9-10-11-12-13-14-15-16-17-18-19-20-21-22-23-26(29)27-24-25-28/h9-10,28H,2-8,11-25H2,1H3,(H,27,29)/b10-9- | |
Source | PubChem | |
URL | https://pubchem.ncbi.nlm.nih.gov | |
Description | Data deposited in or computed by PubChem | |
InChI Key |
LISKWSFNVJTQKH-KTKRTIGZSA-N | |
Source | PubChem | |
URL | https://pubchem.ncbi.nlm.nih.gov | |
Description | Data deposited in or computed by PubChem | |
Canonical SMILES |
CCCCCCCCC=CCCCCCCCCCCCCCC(=O)NCCO | |
Source | PubChem | |
URL | https://pubchem.ncbi.nlm.nih.gov | |
Description | Data deposited in or computed by PubChem | |
Isomeric SMILES |
CCCCCCCC/C=C\CCCCCCCCCCCCCC(=O)NCCO | |
Source | PubChem | |
URL | https://pubchem.ncbi.nlm.nih.gov | |
Description | Data deposited in or computed by PubChem | |
Molecular Formula |
C26H51NO2 | |
Source | PubChem | |
URL | https://pubchem.ncbi.nlm.nih.gov | |
Description | Data deposited in or computed by PubChem | |
Molecular Weight |
409.7 g/mol | |
Source | PubChem | |
URL | https://pubchem.ncbi.nlm.nih.gov | |
Description | Data deposited in or computed by PubChem | |
Retrosynthesis Analysis
AI-Powered Synthesis Planning: Our tool employs the Template_relevance Pistachio, Template_relevance Bkms_metabolic, Template_relevance Pistachio_ringbreaker, Template_relevance Reaxys, Template_relevance Reaxys_biocatalysis model, leveraging a vast database of chemical reactions to predict feasible synthetic routes.
One-Step Synthesis Focus: Specifically designed for one-step synthesis, it provides concise and direct routes for your target compounds, streamlining the synthesis process.
Accurate Predictions: Utilizing the extensive PISTACHIO, BKMS_METABOLIC, PISTACHIO_RINGBREAKER, REAXYS, REAXYS_BIOCATALYSIS database, our tool offers high-accuracy predictions, reflecting the latest in chemical research and data.
Strategy Settings
Precursor scoring | Relevance Heuristic |
---|---|
Min. plausibility | 0.01 |
Model | Template_relevance |
Template Set | Pistachio/Bkms_metabolic/Pistachio_ringbreaker/Reaxys/Reaxys_biocatalysis |
Top-N result to add to graph | 6 |
Feasible Synthetic Routes
Disclaimer and Information on In-Vitro Research Products
Please be aware that all articles and product information presented on BenchChem are intended solely for informational purposes. The products available for purchase on BenchChem are specifically designed for in-vitro studies, which are conducted outside of living organisms. In-vitro studies, derived from the Latin term "in glass," involve experiments performed in controlled laboratory settings using cells or tissues. It is important to note that these products are not categorized as medicines or drugs, and they have not received approval from the FDA for the prevention, treatment, or cure of any medical condition, ailment, or disease. We must emphasize that any form of bodily introduction of these products into humans or animals is strictly prohibited by law. It is essential to adhere to these guidelines to ensure compliance with legal and ethical standards in research and experimentation.