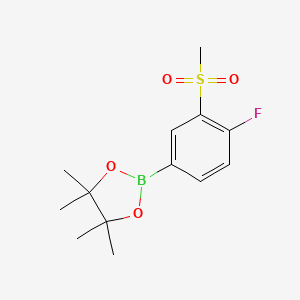
4-Fluoro-3-(methylsulfonyl)phenylboronic acid pinacol ester
Overview
Description
4-Fluoro-3-(methylsulfonyl)phenylboronic acid pinacol ester is a boronic acid derivative where the boron atom is protected as a pinacol ester. The compound features a fluorine atom at the 4-position and a methylsulfonyl group at the 3-position of the phenyl ring. This structure enhances stability and modulates reactivity, making it valuable in Suzuki-Miyaura cross-coupling reactions and responsive material design.
Preparation Methods
Synthetic Routes and Reaction Conditions
The synthesis of 4-Fluoro-3-(methylsulfonyl)phenylboronic acid pinacol ester typically involves the following steps:
Formation of the Boronic Acid Intermediate: The starting material, 4-fluoro-3-(methylsulfonyl)phenylboronic acid, is synthesized through the reaction of 4-fluoro-3-(methylsulfonyl)phenylmagnesium bromide with trimethyl borate, followed by hydrolysis.
Esterification: The boronic acid intermediate is then reacted with pinacol in the presence of a dehydrating agent such as toluene or benzene to form the pinacol ester.
Industrial Production Methods
In industrial settings, the production of this compound follows similar synthetic routes but on a larger scale. The process involves optimized reaction conditions to ensure high yield and purity. Continuous flow reactors and automated systems are often employed to enhance efficiency and scalability.
Chemical Reactions Analysis
Types of Reactions
4-Fluoro-3-(methylsulfonyl)phenylboronic acid pinacol ester undergoes various chemical reactions, including:
Suzuki-Miyaura Cross-Coupling: This reaction involves the coupling of the boronic ester with aryl or vinyl halides in the presence of a palladium catalyst and a base.
Protodeboronation: This reaction involves the removal of the boronic ester group, typically using a protic acid or a base, resulting in the formation of the corresponding aryl or alkyl compound.
Common Reagents and Conditions
Palladium Catalysts: Commonly used in Suzuki-Miyaura cross-coupling reactions.
Bases: Such as potassium carbonate or sodium hydroxide.
Solvents: Toluene, ethanol, or water.
Major Products Formed
Biaryl Compounds: Formed through Suzuki-Miyaura cross-coupling.
Aryl or Alkyl Compounds: Formed through protodeboronation.
Scientific Research Applications
4-Fluoro-3-(methylsulfonyl)phenylboronic acid pinacol ester has numerous applications in scientific research:
Organic Synthesis: Widely used in the synthesis of complex organic molecules, particularly in the pharmaceutical industry for the development of drug candidates.
Material Science: Utilized in the preparation of advanced materials, including polymers and electronic materials.
Biological Research: Employed in the synthesis of biologically active molecules and probes for studying biological processes.
Mechanism of Action
The mechanism of action of 4-Fluoro-3-(methylsulfonyl)phenylboronic acid pinacol ester in Suzuki-Miyaura cross-coupling involves several key steps:
Oxidative Addition: The palladium catalyst undergoes oxidative addition with the aryl or vinyl halide, forming a palladium complex.
Transmetalation: The boronic ester transfers its organic group to the palladium complex.
Reductive Elimination: The palladium complex undergoes reductive elimination, forming the desired biaryl or substituted alkene product.
Comparison with Similar Compounds
Solubility in Organic Solvents
Pinacol esters generally exhibit superior solubility in organic solvents compared to parent boronic acids. For example:
- 4-Fluoro-3-(methylsulfonyl)phenylboronic acid pinacol ester is expected to follow trends observed for other pinacol esters, showing high solubility in polar solvents like chloroform (up to ~0.9 mole fraction at 25°C) and moderate solubility in hydrocarbons .
- Azaesters of phenylboronic acid (e.g., azaester 3) display significant solvent-dependent solubility, with chloroform > 3-pentanone > methylcyclohexane. This contrasts with pinacol esters, which show smaller differences between solvents .
Table 1: Solubility Trends of Boronic Acid Derivatives
Compound | Chloroform | 3-Pentanone | Methylcyclohexane |
---|---|---|---|
Phenylboronic acid | Moderate | High | Very Low |
Pinacol ester (generic) | High | High | Low |
Azaester | Very High | Moderate | Very Low |
4-Fluoro-3-(methylsulfonyl)phenyl* | High (est.) | High (est.) | Low (est.) |
*Estimated based on pinacol ester trends .
Performance in Responsive Material Systems
Pinacol esters are widely incorporated into polymers for stimuli-responsive behavior:
- For instance, HPAP-based micelles degrade rapidly under H₂O₂ exposure, while sulfonyl groups might require stronger oxidative conditions .
- Dual pH/ROS Responsiveness: Copolymers combining pinacol esters with acid-labile groups (e.g., acetals) enable dual-responsive nanoparticles. The target compound’s fluorine atom could enhance stability in acidic environments, prolonging circulation time .
Biological Activity
4-Fluoro-3-(methylsulfonyl)phenylboronic acid pinacol ester (CAS No. 1203655-90-6) is a boronic acid derivative that has garnered attention in medicinal chemistry due to its potential biological activities, particularly in the fields of oncology and infectious diseases. This compound is characterized by its unique molecular structure, which includes a fluorine atom and a methylsulfonyl group, contributing to its reactivity and biological interactions.
- Molecular Formula : C₁₃H₁₈BFO₄S
- Molecular Weight : 300.15 g/mol
- CAS Number : 1203655-90-6
The biological activity of this compound is primarily attributed to its ability to interact with various biological targets, including enzymes and receptors. Boronic acids are known for their ability to form reversible covalent bonds with diols, which may enhance their efficacy in targeting specific biomolecules.
Biological Activity
Research indicates that boronic acid derivatives exhibit a range of biological activities, including:
- Anticancer Activity : Compounds similar to 4-Fluoro-3-(methylsulfonyl)phenylboronic acid have been shown to inhibit proteasome activity, leading to cell cycle arrest and apoptosis in cancer cells. For instance, studies have demonstrated that certain boronic acids can effectively inhibit the growth of multiple myeloma cells by modulating the proteasome pathway .
- Antibacterial Properties : Boronic acids have been explored for their potential as antibacterial agents. The mechanism often involves the inhibition of β-lactamases, enzymes that confer resistance to β-lactam antibiotics. Research has shown that boronic acid derivatives can act as effective inhibitors against these enzymes, thereby restoring the efficacy of existing antibiotics .
- Antiviral Activity : Some studies suggest that boronic acids can interfere with viral replication mechanisms, although specific data on this compound remains limited in this regard.
Table 1: Summary of Biological Activities
Case Study: Anticancer Efficacy
A study published in Scientific Reports evaluated the anticancer properties of various boronic acid derivatives, including those similar to 4-Fluoro-3-(methylsulfonyl)phenylboronic acid. The research demonstrated significant inhibition of cell proliferation in multiple myeloma cell lines, with IC50 values indicating potent activity against cancerous cells. The mechanism was linked to proteasome inhibition, leading to cell cycle arrest at the G2/M phase .
Case Study: Antibacterial Action
In another study focusing on bacterial resistance mechanisms, researchers investigated the efficacy of boronic acids against resistant strains of bacteria. The findings revealed that compounds like 4-Fluoro-3-(methylsulfonyl)phenylboronic acid could effectively inhibit β-lactamases, thus enhancing the activity of β-lactam antibiotics against resistant bacterial strains .
Q & A
Basic Research Questions
Q. What is the role of the pinacol ester group in stabilizing 4-Fluoro-3-(methylsulfonyl)phenylboronic acid during Suzuki-Miyaura cross-coupling reactions?
The pinacol ester protects the boronic acid from hydrolysis and protodeboronation, enhancing shelf stability and enabling controlled reactivity. In Suzuki couplings, the ester is cleaved in situ under basic conditions (e.g., K₂CO₃ or CsF) to release the active boronic acid. This mechanism prevents premature decomposition, particularly in moisture-sensitive reactions .
Q. How do the electron-withdrawing substituents (fluoro and methylsulfonyl) influence the compound’s electronic properties and reactivity?
The fluorine and methylsulfonyl groups withdraw electron density via inductive effects, polarizing the aromatic ring and activating specific positions for nucleophilic or electrophilic attacks. This electronic modulation enhances regioselectivity in cross-couplings, favoring reactions with electron-deficient aryl halides. Computational studies (e.g., DFT) can predict charge distribution, validated experimentally by NMR coupling constants .
Q. Which analytical techniques are most effective for characterizing this compound and confirming purity?
- ¹H/¹³C NMR : Aromatic protons (δ 7.2–8.1 ppm) and pinacol methyl groups (δ 1.0–1.3 ppm) confirm structural integrity.
- HPLC-UV : Reverse-phase C18 columns with methanol/water gradients assess purity (>98%).
- Mass Spectrometry (HRMS) : Validates molecular weight (e.g., [M+H]⁺ calculated for C₁₃H₁₇BFSO₄: 314.08) .
Q. What are the standard storage conditions to prevent degradation of this boronic ester?
Store at 2–8°C under inert gas (Ar/N₂) in airtight containers. Desiccants (e.g., molecular sieves) prevent hydrolysis. Avoid prolonged exposure to light or humidity, as the methylsulfonyl group may degrade under acidic conditions .
Q. How is this compound typically used in medicinal chemistry scaffold synthesis?
It serves as a key intermediate for introducing fluorine and sulfone motifs into bioactive molecules. For example, it can couple with heteroaryl halides to generate kinase inhibitors or protease-resistant analogs. Reaction yields are optimized using Pd(OAc)₂/XPhos catalysts in THF/H₂O (3:1) at 60°C .
Advanced Research Questions
Q. How can researchers resolve contradictions in catalytic system performance when coupling with sterically hindered aryl halides?
Contradictory results often arise from ligand steric effects and solvent polarity. Systematic optimization includes:
- Testing bulky ligands (e.g., SPhos or RuPhos) to reduce steric clashes.
- Switching to polar aprotic solvents (DME or DMF) to improve solubility.
- Pre-activating the boronic ester with BF₃·OEt₂ to enhance transmetallation kinetics .
Parameter | Optimization Strategy | Expected Outcome |
---|---|---|
Ligand | SPhos (vs. PPh₃) | +20–30% yield |
Solvent | DME/H₂O (vs. THF) | Improved miscibility |
Pre-activation | BF₃·OEt₂ | Faster kinetics |
Q. What methodologies address low reproducibility in cross-coupling yields across laboratories?
Standardize protocols for:
- Moisture control : Use gloveboxes or Schlenk lines to exclude water.
- Catalyst preformation : Pre-mix Pd catalysts with ligands in degassed solvents.
- Base selection : Test alternatives (e.g., K₃PO₄ vs. Cs₂CO₃) to match substrate solubility .
Q. How do competing reaction pathways (kinetic vs. thermodynamic control) affect product distribution in multi-component couplings?
Kinetic control favors the faster-forming isomer (e.g., ortho-substituted products), while thermodynamic control stabilizes the more stable isomer (e.g., para-substituted). Monitor time-dependent product ratios via LC-MS and adjust reaction duration or temperature accordingly. For example, shorter reaction times (1–2 h) at 80°C favor kinetic products .
Q. What advanced techniques validate the compound’s interaction with biological targets (e.g., proteasomes)?
- Surface Plasmon Resonance (SPR) : Measures binding affinity (KD) to immobilized proteins.
- X-ray Crystallography : Resolves binding modes of boronate-protein adducts.
- Cellular Assays : Evaluate apoptosis via flow cytometry after proteasome inhibition .
Q. How can computational tools predict the compound’s reactivity in novel reaction systems?
- DFT Calculations : Model transition states to predict regioselectivity in cross-couplings.
- Molecular Dynamics (MD) : Simulate solvent effects on reaction pathways.
- Machine Learning : Train models on existing Suzuki reaction datasets to forecast optimal conditions .
Q. Data Contradiction Analysis
Case Study : Discrepancies in reported yields for couplings with electron-rich aryl halides.
Properties
IUPAC Name |
2-(4-fluoro-3-methylsulfonylphenyl)-4,4,5,5-tetramethyl-1,3,2-dioxaborolane | |
---|---|---|
Details | Computed by Lexichem TK 2.7.0 (PubChem release 2021.05.07) | |
Source | PubChem | |
URL | https://pubchem.ncbi.nlm.nih.gov | |
Description | Data deposited in or computed by PubChem | |
InChI |
InChI=1S/C13H18BFO4S/c1-12(2)13(3,4)19-14(18-12)9-6-7-10(15)11(8-9)20(5,16)17/h6-8H,1-5H3 | |
Details | Computed by InChI 1.0.6 (PubChem release 2021.05.07) | |
Source | PubChem | |
URL | https://pubchem.ncbi.nlm.nih.gov | |
Description | Data deposited in or computed by PubChem | |
InChI Key |
PIZBTEXZBRHRIA-UHFFFAOYSA-N | |
Details | Computed by InChI 1.0.6 (PubChem release 2021.05.07) | |
Source | PubChem | |
URL | https://pubchem.ncbi.nlm.nih.gov | |
Description | Data deposited in or computed by PubChem | |
Canonical SMILES |
B1(OC(C(O1)(C)C)(C)C)C2=CC(=C(C=C2)F)S(=O)(=O)C | |
Details | Computed by OEChem 2.3.0 (PubChem release 2021.05.07) | |
Source | PubChem | |
URL | https://pubchem.ncbi.nlm.nih.gov | |
Description | Data deposited in or computed by PubChem | |
Molecular Formula |
C13H18BFO4S | |
Details | Computed by PubChem 2.1 (PubChem release 2021.05.07) | |
Source | PubChem | |
URL | https://pubchem.ncbi.nlm.nih.gov | |
Description | Data deposited in or computed by PubChem | |
Molecular Weight |
300.2 g/mol | |
Details | Computed by PubChem 2.1 (PubChem release 2021.05.07) | |
Source | PubChem | |
URL | https://pubchem.ncbi.nlm.nih.gov | |
Description | Data deposited in or computed by PubChem | |
Retrosynthesis Analysis
AI-Powered Synthesis Planning: Our tool employs the Template_relevance Pistachio, Template_relevance Bkms_metabolic, Template_relevance Pistachio_ringbreaker, Template_relevance Reaxys, Template_relevance Reaxys_biocatalysis model, leveraging a vast database of chemical reactions to predict feasible synthetic routes.
One-Step Synthesis Focus: Specifically designed for one-step synthesis, it provides concise and direct routes for your target compounds, streamlining the synthesis process.
Accurate Predictions: Utilizing the extensive PISTACHIO, BKMS_METABOLIC, PISTACHIO_RINGBREAKER, REAXYS, REAXYS_BIOCATALYSIS database, our tool offers high-accuracy predictions, reflecting the latest in chemical research and data.
Strategy Settings
Precursor scoring | Relevance Heuristic |
---|---|
Min. plausibility | 0.01 |
Model | Template_relevance |
Template Set | Pistachio/Bkms_metabolic/Pistachio_ringbreaker/Reaxys/Reaxys_biocatalysis |
Top-N result to add to graph | 6 |
Feasible Synthetic Routes
Disclaimer and Information on In-Vitro Research Products
Please be aware that all articles and product information presented on BenchChem are intended solely for informational purposes. The products available for purchase on BenchChem are specifically designed for in-vitro studies, which are conducted outside of living organisms. In-vitro studies, derived from the Latin term "in glass," involve experiments performed in controlled laboratory settings using cells or tissues. It is important to note that these products are not categorized as medicines or drugs, and they have not received approval from the FDA for the prevention, treatment, or cure of any medical condition, ailment, or disease. We must emphasize that any form of bodily introduction of these products into humans or animals is strictly prohibited by law. It is essential to adhere to these guidelines to ensure compliance with legal and ethical standards in research and experimentation.