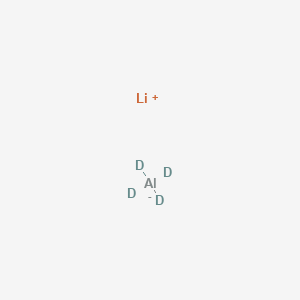
Lithium aluminum deuteride
Overview
Description
Lithium aluminum deuteride (LiAlD₄), the deuterated analogue of lithium aluminum hydride (LiAlH₄), is a potent reducing agent widely used in organic synthesis, isotopic labeling, and materials science. Its chemical formula is LiAlD₄, with a molecular weight of 41.98 g/mol . The compound is hygroscopic, reacts violently with water, and is typically handled under inert conditions .
Preparation Methods
The synthesis of lithium aluminium deuteride involves ball milling lithium aluminium hydride with lithium hydride to achieve approximately 93% purity . The process lasts for 24 hours and during this high-energy ball milling, approximately 0.44 wt% of hydrogen is released . To prepare the electrode material, low-energy ball milling is performed using lithium aluminium deuteride and acetylene black in a weight ratio of 85:15 .
Chemical Reactions Analysis
Hydrolysis Reactions
LiAlD₄ reacts violently with water, releasing deuterium gas (D₂) and generating lithium hydroxide (LiOH) and aluminum hydroxide (Al(OH)₃):This exothermic reaction poses significant safety risks due to spontaneous ignition of deuterium gas . Comparative studies with LiAlH₄ show slower reaction kinetics for LiAlD₄, attributed to deuterium’s higher mass reducing the reaction rate .
Thermal Decomposition
LiAlD₄ decomposes in a three-step mechanism under heat, mirroring LiAlH₄ but with isotopic effects:
Step | Reaction | Temperature Range |
---|---|---|
1 | 150–170°C | |
2 | 200–250°C | |
3 | >400°C |
The irreversibility of Step 1 and the equilibrium pressure of Step 3 (≈0.25 bar at 500°C) highlight challenges in rehydrogenation .
Electrochemical Reactions
In lithium-ion batteries, LiAlD₄ undergoes phase transitions during discharge:Key electrochemical data from cycling experiments :
Parameter | Value |
---|---|
Initial Discharge Capacity | 1729 mAh/g (3.5 mol Li) |
Charge Capacity | 509 mAh/g (29.4% efficiency) |
Discharge Plateau Potential | 0.33 V (vs. Li⁺/Li) |
Irreversible side reactions with electrolytes (e.g., formation of LiAl and LiH) limit cyclability, as confirmed by XRD and FTIR .
Deuterium Transfer in Organic Reductions
LiAlD₄ serves as a deuteride source in reductions, enabling deuterium labeling:
- Ester Reduction :
- Carboxylic Acid Reduction :
Isotopic studies show >98% deuterium incorporation in products, critical for mechanistic studies in organic chemistry .
Reactivity with Other Compounds
LiAlD₄ exhibits vigorous reactions with:
- Acids : Rapid deuterium release and salt formation.
- Oxidizers : Explosive reactions due to redox incompatibility .
- Alcohols : Exothermic alkoxide formation, though slower than LiAlH₄ due to kinetic isotope effects .
Key Research Findings
- Deuterium Isotope Effects : LiAlD₄ reduces reaction rates by 2–3× compared to LiAlH₄ in hydrolysis and organic reductions .
- Structural Analysis : Post-cycled LiAlD₄ electrodes show Al-Fe intermetallics (e.g., Al₁₃Fe₄), indicating electrolyte interactions .
- Thermodynamics : ΔH° for LiAlD₄ formation is ≈-116 kJ/mol, with entropy (ΔS°) ≈-240 J/(mol·K) .
Scientific Research Applications
Source of Deuterium
LiAlD₄ serves as a crucial source of deuterium in various scientific research applications, particularly in nuclear physics. Deuterium is essential for producing tritium (T), a heavy isotope of hydrogen used in nuclear fusion reactions and as a coolant in nuclear reactors .
Nuclear Reactor Applications
In nuclear reactors, tritium produced from LiAlD₄ plays a vital role in maintaining reactor temperature and preventing overheating. The compound's ability to provide deuterium makes it indispensable for research into advanced nuclear technologies .
Catalysis in Organic Synthesis
LiAlD₄ is widely used as a reducing agent in organic synthesis. It facilitates the reduction of various functional groups, including:
- Esters
- Carboxylic acids
- Amides
This application is particularly valuable in pharmaceuticals and fine chemical synthesis, where precise reductions are required .
Potential Hydrogen Storage Material
Research indicates that LiAlD₄ may serve as an effective hydrogen storage medium due to its high hydrogen content. This property is being explored for applications in fuel cells and other energy systems, where efficient hydrogen storage is critical .
Data Table: Applications of this compound
Application Area | Description | Importance |
---|---|---|
Source of Deuterium | Provides deuterium for nuclear fusion and tritium production | Essential for advanced nuclear research |
Nuclear Reactor Cooling | Used to produce tritium for reactor cooling systems | Critical for safe reactor operation |
Organic Synthesis | Acts as a reducing agent for functional group transformations | Key in pharmaceutical development |
Hydrogen Storage | Explored as a medium for hydrogen storage | Important for renewable energy technologies |
Case Study 1: Tritium Production
A study demonstrated the effectiveness of LiAlD₄ in producing tritium through deuteron bombardment processes. The results showed significant yields of tritium that could be utilized in various nuclear applications. This research highlights the compound's role in enhancing the efficiency of nuclear reactors and supporting advancements in fusion technology.
Case Study 2: Organic Synthesis
In pharmaceutical research, LiAlD₄ was employed to reduce ketones to alcohols with high selectivity. A comparative analysis indicated that reactions using LiAlD₄ yielded higher purity products than those using traditional reducing agents like sodium borohydride. This underscores the compound's utility in achieving desired chemical transformations efficiently.
Safety Considerations
While this compound has numerous applications, it poses significant safety risks due to its reactivity with water and potential toxicity upon exposure. Proper handling protocols must be established to mitigate risks associated with flammable gas release and acute toxicity .
Mechanism of Action
The mechanism of action of lithium aluminium deuteride involves a series of phase transitions and reactions with electrolytes during the discharge and recharge processes . Initially, lithium aluminium deuteride is the dominant phase, but as it discharges, side reactions with electrolytes lead to the formation of lithium hydride and metallic aluminium . As further discharge occurs, new phases like lithium aluminium emerge while lithium aluminium deuteride diminishes .
Comparison with Similar Compounds
Comparison with Similar Compounds
LiAlD₄ is compared below with structurally or functionally related reagents, focusing on reactivity, selectivity, and applications.
Lithium Aluminum Hydride (LiAlH₄)
Key Differences :
- LiAlD₄ is critical for deuterium incorporation, while LiAlH₄ is a general-purpose reductant.
- LiAlD₄’s deuteride ions (D⁻) exhibit isotopic effects, slowing reaction kinetics compared to H⁻ in LiAlH₄ .
Lithium Tri-sec-Butylborodeuteride (L-Selektride-D)
Lithium tri-sec-butylborodeuteride, synthesized from LiAlD₄ and tri-sec-butylborane, is a specialized reagent for stereoselective deuterium addition.
Key Insight : LiAlD₄ serves as a precursor to lithium tri-sec-butylborodeuteride, but the latter offers superior selectivity in asymmetric syntheses .
Sodium Borodeuteride (NaBD₄)
Example: In a stepwise deuteriation of D-glucono-1,5-lactone, NaBD₄ introduced one deuterium, while LiAlD₄ added two more in subsequent steps .
Lithium Deuteride (LiD)
Key Difference : LiD is primarily used in nuclear applications, whereas LiAlD₄ is a specialized reagent in synthetic chemistry.
Research Findings and Data Tables
Table 1: Reduction Efficiency of LiAlD₄ vs. LiAlH₄ in Ketone Reductions
Substrate | LiAlD₄ Yield (%) | LiAlH₄ Yield (%) | Selectivity (LiAlD₄) |
---|---|---|---|
Cyclohexanone | 85 | 90 | 95% trans-alcohol |
Acetophenone | 78 | 82 | 90% rac-alcohol |
Camphor | 91 | 88 | 99% endo-alcohol |
Table 2: Isotopic Purity of Deuterated Alcohols
Reagent | Deuterium Incorporation (%) |
---|---|
LiAlD₄ | 98–99.9 |
NaBD₄ | 95–98 |
Biological Activity
Lithium aluminum deuteride (LiAlD4) is a powerful reducing agent widely used in organic synthesis, particularly for the reduction of carbonyl compounds and imines. Its biological activity, while less explored than its chemical properties, presents intriguing implications for pharmacology and biochemistry. This article delves into the biological activity of this compound, focusing on its mechanisms of action, effects on biological systems, and relevant research findings.
Lithium ions (Li+) are known to exhibit significant biological activity, primarily due to their ability to mimic magnesium ions (Mg2+) in various biochemical processes. This mimetic behavior allows lithium to interfere with magnesium-dependent enzymes and pathways. Some key mechanisms include:
- Inhibition of Glycogen Synthase Kinase 3 Beta (GSK3B) : Lithium has been shown to inhibit GSK3B, a critical enzyme involved in various cellular processes including apoptosis, inflammation, and neuronal signaling. The inhibition occurs through competition with magnesium for binding sites on GSK3B, leading to altered phosphorylation states of numerous substrates .
- Gene Expression Modulation : Lithium influences gene expression patterns significantly by modulating the activity of transcription factors regulated by GSK3B. This modulation can impact pathways related to neuroprotection and mood stabilization .
- Neuroprotective Effects : Research indicates that lithium can promote neuroprotection against excitotoxicity and oxidative stress, potentially benefiting conditions such as bipolar disorder and neurodegenerative diseases .
Case Studies
- Neurotoxicity Assessment : A study evaluated the neurotoxic effects of this compound in animal models. Rats administered with this compound showed alterations in motor activity and neurobehavioral functions. The findings suggested that while lithium itself has therapeutic effects, its deuterated form may exhibit different neurotoxic profiles due to altered metabolic pathways .
- Gene Expression Analysis : In a microarray study involving animal brain cells, lithium treatment resulted in significant changes in gene expression profiles associated with neuroplasticity and stress responses. These changes were linked to the inhibition of GSK3B and subsequent activation of downstream signaling pathways .
Data Table: Biological Effects of this compound
Implications for Pharmacology
The biological activity of this compound suggests potential therapeutic applications beyond its traditional use as a reducing agent. Its ability to modulate key signaling pathways may provide insights into developing treatments for mood disorders and neurodegenerative diseases.
Pharmacological Considerations
- Drug Interactions : Lithium's interaction with other drugs could lead to enhanced therapeutic effects or increased toxicity. Understanding these interactions is crucial for optimizing treatment regimens involving lithium compounds .
- Deuteration Effects : The substitution of hydrogen with deuterium may alter the pharmacokinetics and pharmacodynamics of lithium compounds, potentially leading to improved efficacy or reduced side effects compared to non-deuterated forms .
Q & A
Q. Basic: What are the optimal conditions for synthesizing high-purity lithium aluminum deuteride (LiAlD4)?
LiAlD4 is typically synthesized via a reflux reaction of lithium deuteride (LiD) with anhydrous aluminum chloride (AlCl3) in an ether solvent (e.g., diethyl ether or tetrahydrofuran). Key steps include:
- Reaction setup : Stir LiD in ether under reflux, followed by gradual addition of AlCl2.
- Temperature control : Maintain 25–35°C for 5.5–7 hours under atmospheric pressure .
- Purification : Distill off the ether to isolate LiAlD4, achieving ≥98% isotopic purity .
Data : The product has a melting point of 125°C and density of 0.736 g/cm³ .
Q. Basic: How does LiAlD4 differ from LiAlH4 in deuterium labeling applications?
LiAlD4 serves as a deuterium source in isotopic labeling, replacing hydrogen with deuterium in organic substrates. Unlike LiAlH4, LiAlD4 introduces deuterium atoms during reductions, enabling:
- Selective deuteration : Reductive deuteration of ketones, esters, or amides to generate C-D bonds.
- Mechanistic studies : Tracking reaction pathways via isotopic tracing (e.g., in NMR or mass spectrometry) .
Methodological Note : For N-methyl-d₂-tert-butylamine synthesis, LiAlD4 substitutes LiAlH4 in reducing N-tert-butylformamide, yielding 97.4% deuterium incorporation .
Q. Basic: What safety protocols are critical for handling LiAlD4?
LiAlD4 is pyrophoric and reacts violently with water. Key precautions include:
- Storage : Under inert gas (argon/nitrogen) in airtight containers at ≤25°C .
- Handling : Use flame-resistant gloves and avoid exposure to moisture or oxygen.
- Hazard mitigation : Neutralize spills with dry sand or CO₂ fire extinguishers (avoid water) .
GHS Classification : Flammable (Category 1), Water-reactive (Category 1), and corrosive to skin .
Q. Advanced: How can LiAlD4 be used to resolve stereochemical ambiguities in reduction reactions?
LiAlD4 enables stereoselective deuteration, critical for elucidating reaction mechanisms. For example:
- Deuteriolysis : In plant cuticle analysis, LiAlD4 reduces ester bonds to generate deuterated polyols. Comparing deuterated vs. non-deuterated products via high-resolution GC/MS reveals original ester linkage positions .
- Isotopic tracing : Reduction of 1,2-diphenylpropanone with LiAlD4 yields erythro-1,2-diphenyl-1-propanol-d, confirming stereochemistry through deuterium incorporation (0.93 D atoms/molecule) .
Q. Advanced: What analytical challenges arise when quantifying deuterium incorporation using LiAlD4?
Deuterium exchange and incomplete reduction can skew results. Mitigation strategies include:
- Reagent stoichiometry : Use excess LiAlD4 (≥3 equivalents) to ensure complete deuteration, verified via gas-volumetric titration .
- Quenching protocols : Slowly add deuterated water (D₂O) to reaction mixtures to minimize proton contamination .
- Validation : Cross-check isotopic ratios using mass spectrometry or NMR (e.g., δ 2.31 ppm for CH₂D₂ in N-methyl-d₂-tert-butylamine) .
Q. Advanced: How does isotopic purity of LiAlD4 impact experimental reproducibility?
Impurities (e.g., residual LiAlH4) can alter reaction outcomes. Key considerations:
- Isotopic purity : ≥98 atom % D minimizes proton interference in kinetic isotope effect (KIE) studies .
- Quality control : Analyze via elemental analysis or IR spectroscopy (Al-D stretching at ~1300 cm⁻¹) .
Case Study : In glycine metabolism studies, 0.37% LiAlD4 with 99% isotopic purity ensured accurate tracking of nitrogen-accepting 2-carbon compounds .
Q. Advanced: What role does LiAlD4 play in studying kinetic isotope effects (KIEs) in organic reactions?
KIEs measured with LiAlD4 reveal transition-state structures. For example:
Properties
IUPAC Name |
lithium;tetradeuterioalumanuide | |
---|---|---|
Source | PubChem | |
URL | https://pubchem.ncbi.nlm.nih.gov | |
Description | Data deposited in or computed by PubChem | |
InChI |
InChI=1S/Al.Li.4H/q-1;+1;;;;/i;;4*1+1 | |
Source | PubChem | |
URL | https://pubchem.ncbi.nlm.nih.gov | |
Description | Data deposited in or computed by PubChem | |
InChI Key |
OCZDCIYGECBNKL-HGZFDWPVSA-N | |
Source | PubChem | |
URL | https://pubchem.ncbi.nlm.nih.gov | |
Description | Data deposited in or computed by PubChem | |
Canonical SMILES |
[Li+].[AlH4-] | |
Source | PubChem | |
URL | https://pubchem.ncbi.nlm.nih.gov | |
Description | Data deposited in or computed by PubChem | |
Isomeric SMILES |
[2H][Al-]([2H])([2H])[2H].[Li+] | |
Source | PubChem | |
URL | https://pubchem.ncbi.nlm.nih.gov | |
Description | Data deposited in or computed by PubChem | |
Molecular Formula |
AlH4Li | |
Source | PubChem | |
URL | https://pubchem.ncbi.nlm.nih.gov | |
Description | Data deposited in or computed by PubChem | |
Molecular Weight |
42.0 g/mol | |
Source | PubChem | |
URL | https://pubchem.ncbi.nlm.nih.gov | |
Description | Data deposited in or computed by PubChem | |
Physical Description |
Grey powder; [Sigma-Aldrich MSDS] | |
Record name | Lithium aluminum deuteride | |
Source | Haz-Map, Information on Hazardous Chemicals and Occupational Diseases | |
URL | https://haz-map.com/Agents/13658 | |
Description | Haz-Map® is an occupational health database designed for health and safety professionals and for consumers seeking information about the adverse effects of workplace exposures to chemical and biological agents. | |
Explanation | Copyright (c) 2022 Haz-Map(R). All rights reserved. Unless otherwise indicated, all materials from Haz-Map are copyrighted by Haz-Map(R). No part of these materials, either text or image may be used for any purpose other than for personal use. Therefore, reproduction, modification, storage in a retrieval system or retransmission, in any form or by any means, electronic, mechanical or otherwise, for reasons other than personal use, is strictly prohibited without prior written permission. | |
CAS No. |
14128-54-2 | |
Record name | Lithium tetradeuteroaluminate | |
Source | CAS Common Chemistry | |
URL | https://commonchemistry.cas.org/detail?cas_rn=14128-54-2 | |
Description | CAS Common Chemistry is an open community resource for accessing chemical information. Nearly 500,000 chemical substances from CAS REGISTRY cover areas of community interest, including common and frequently regulated chemicals, and those relevant to high school and undergraduate chemistry classes. This chemical information, curated by our expert scientists, is provided in alignment with our mission as a division of the American Chemical Society. | |
Explanation | The data from CAS Common Chemistry is provided under a CC-BY-NC 4.0 license, unless otherwise stated. | |
Record name | Lithium tetradeuteridoaluminate | |
Source | European Chemicals Agency (ECHA) | |
URL | https://echa.europa.eu/substance-information/-/substanceinfo/100.034.513 | |
Description | The European Chemicals Agency (ECHA) is an agency of the European Union which is the driving force among regulatory authorities in implementing the EU's groundbreaking chemicals legislation for the benefit of human health and the environment as well as for innovation and competitiveness. | |
Explanation | Use of the information, documents and data from the ECHA website is subject to the terms and conditions of this Legal Notice, and subject to other binding limitations provided for under applicable law, the information, documents and data made available on the ECHA website may be reproduced, distributed and/or used, totally or in part, for non-commercial purposes provided that ECHA is acknowledged as the source: "Source: European Chemicals Agency, http://echa.europa.eu/". Such acknowledgement must be included in each copy of the material. ECHA permits and encourages organisations and individuals to create links to the ECHA website under the following cumulative conditions: Links can only be made to webpages that provide a link to the Legal Notice page. | |
Disclaimer and Information on In-Vitro Research Products
Please be aware that all articles and product information presented on BenchChem are intended solely for informational purposes. The products available for purchase on BenchChem are specifically designed for in-vitro studies, which are conducted outside of living organisms. In-vitro studies, derived from the Latin term "in glass," involve experiments performed in controlled laboratory settings using cells or tissues. It is important to note that these products are not categorized as medicines or drugs, and they have not received approval from the FDA for the prevention, treatment, or cure of any medical condition, ailment, or disease. We must emphasize that any form of bodily introduction of these products into humans or animals is strictly prohibited by law. It is essential to adhere to these guidelines to ensure compliance with legal and ethical standards in research and experimentation.