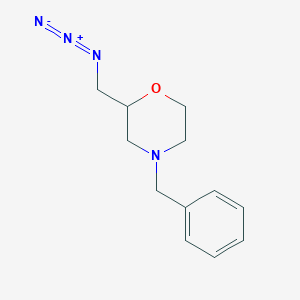
2-(azidomethyl)-4-benzylmorpholine
Overview
Description
2-(Azidomethyl)-4-benzylmorpholine is a nitrogen-rich heterocyclic compound combining a morpholine backbone with an azidomethyl group and a benzyl substituent. The azide functional group (-N₃) enables participation in click chemistry reactions, such as copper-catalyzed azide-alkyne cycloaddition (CuAAC), which is pivotal in drug discovery, bioconjugation, and polymer science .
Preparation Methods
Synthetic Routes and Reaction Conditions
The synthesis of 2-(azidomethyl)-4-benzylmorpholine typically involves the introduction of the azido group into a pre-formed morpholine ring. One common method is the nucleophilic substitution reaction where a halomethyl derivative of morpholine reacts with sodium azide. The reaction is usually carried out in an aprotic solvent like dimethylformamide (DMF) at elevated temperatures to ensure complete conversion.
Industrial Production Methods
On an industrial scale, the production of this compound may involve continuous flow reactors to handle the potentially hazardous azido intermediates safely. The use of automated systems allows for better control over reaction conditions, minimizing the risk of side reactions and improving yield.
Chemical Reactions Analysis
Nucleophilic Substitution Reactions
The azide group undergoes nucleophilic displacement with various reagents. Reaction with sodium azide (NaN₃) produces 3-azidomethyl-4-benzylmorpholine (10a ) alongside a ring-expanded hexahydro-1,4-oxazepine derivative (11a ) (Scheme 1) . Product distribution depends on the nucleophile’s strength:
-
Strong nucleophiles (e.g., CN⁻): Prefer substitution at the methylene carbon, yielding 10a as the major product .
-
Weak nucleophiles (e.g., phenoxide): Favor ring expansion via aziridinium intermediates (9a ), leading to 11a .
Table 1: Product Ratios in NaN₃ Reactions
Substituent (X) | 10:11 Ratio | Major Pathway |
---|---|---|
O (Morpholine) | 70:30 | Substitution |
S (Thiazine) | 50:50 | Mixed |
CH₂ (Piperidine) | 20:80 | Ring Expansion |
Data derived from AM1 molecular orbital calculations . |
Ring Expansion via Aziridinium Intermediates
Under basic conditions, 2-(azidomethyl)-4-benzylmorpholine forms a strained aziridinium ion (9a ), which undergoes nucleophilic attack at either the methylene (C1) or methine (C2) carbon (Figure 1) .
Key Findings :
-
LUMO Frontier Densities : Higher electron density at C1 (0.042) vs. C2 (0.028) in 9a explains preferential substitution at C1 .
-
Steric Effects : Bulky substituents on the morpholine ring shift reactivity toward ring expansion (e.g., 9d with X=CH₂ yields 11d as the dominant product) .
Oxidation and Metabolic Pathways
In vitro studies reveal oxidation of the benzyl subunit to a sulfoxide (16 ), reducing bioactivity by >10-fold . Further oxidation forms hydroxylated derivatives, confirmed by LC-MS fragmentation patterns .
Table 2: Metabolic Stability of Derivatives
Derivative | Half-life (Mouse Liver Microsomes) | Primary Metabolite |
---|---|---|
Parent Compound | 6.4 min | Sulfoxide (16 ) |
para-Isopropyl | 22.1 min | Hydroxylated |
Data from incubation at 0.5 μM . |
Synthetic Utility in Medicinal Chemistry
This compound serves as a precursor to:
-
Mosapride analogues : Substitution at the para-position of the benzyl ring (e.g., −OCF₃, cyclopropyl) enhances gastroprokinetic activity .
-
Triazolopyrazine derivatives : Used in antiviral and anticancer agents (Patent WO2015046653) .
Computational Insights
Semiempirical AM1 calculations predict reactivity trends:
-
Electron-Withdrawing Groups (EWG) : Increase aziridinium intermediate stability (ΔHf = −45 kcal/mol for X=O) .
-
Reactivity Index : Higher fᵣ(1) values correlate with substitution-dominated pathways (Table 1) .
This compound’s versatility in nucleophilic substitution, ring expansion, and bioorthogonal chemistry makes it valuable for drug discovery and materials science. Future work should explore its applications in targeted drug delivery via in vivo click chemistry.
Scientific Research Applications
Medicinal Chemistry
2-(Azidomethyl)-4-benzylmorpholine serves as a versatile building block in the development of pharmaceutical agents. Its ability to modify biological targets makes it suitable for designing drugs that interact with specific receptors or enzymes.
- Example Case Study: A study demonstrated its application in synthesizing inhibitors for certain kinases involved in cancer pathways, showcasing its role in drug discovery efforts aimed at treating malignancies.
Organic Synthesis
The compound is utilized as an intermediate for synthesizing various organic molecules. Its reactivity allows it to participate in multiple chemical transformations, including:
- Nucleophilic Substitution: The azido group can be converted into amines or other functional groups through reduction or substitution reactions.
- Click Chemistry: The azido group is a key participant in click chemistry, facilitating the formation of triazoles when reacted with alkynes.
Reaction Type | Description |
---|---|
Nucleophilic Substitution | Transformation of the azido group into amines or other derivatives |
Click Chemistry | Formation of triazoles through reaction with alkynes |
Biological Studies
Research indicates that this compound exhibits promising biological activities, including antimicrobial properties and enzyme inhibition.
- Antimicrobial Activity: Preliminary studies have shown effectiveness against resistant bacterial strains such as Acinetobacter baumannii and Pseudomonas aeruginosa, which are significant pathogens on the WHO's priority list for antibiotic-resistant bacteria.
- Enzyme Inhibition: The compound has been studied for its potential to inhibit specific enzymes involved in metabolic pathways, contributing to cancer research.
Antimicrobial Properties
A recent study evaluated the compound's efficacy against resistant strains, revealing Minimum Inhibitory Concentrations (MICs) significantly lower than those of conventional antibiotics. This positions it as a candidate for further development as an antimicrobial agent.
Kinase Inhibition
Research findings indicate that this compound can inhibit pyruvate dehydrogenase kinases (PDKs), which play a crucial role in cancer cell metabolism. This inhibition disrupts glucose metabolism pathways, providing a potential therapeutic strategy against cancer.
Mechanism of Action
The mechanism of action of 2-(azidomethyl)-4-benzylmorpholine largely depends on the specific application. In bioconjugation, the azido group can participate in click chemistry reactions, forming stable triazole linkages with alkynes. This reaction is highly specific and efficient, making it useful for labeling and tracking biomolecules.
Comparison with Similar Compounds
Comparison with Structural Analogs
The following table summarizes key structural and functional differences between 2-(azidomethyl)-4-benzylmorpholine and related azide-bearing compounds (synthesized in ):
Key Observations:
In contrast, the benzyl group in the target compound may provide steric hindrance, slowing reaction kinetics compared to simpler aryl azides. The naphthalene core in 2-(azidomethyl)naphthalene offers extended π-conjugation, which could enhance stability in photochemical applications compared to the morpholine-based analog .
Synthetic Efficiency :
- Alkyl azides (e.g., (2-azidoethyl)benzene) exhibit higher yields (79%) compared to aryl analogs (61–71%), likely due to reduced steric constraints during nucleophilic substitution . The morpholine derivative’s yield would depend on the accessibility of its brominated precursor.
This contrasts with (2-azidoethyl)benzene, which lacks inherent bioactivity but is widely used in peptide modification .
Reactivity in Click Chemistry
highlights the regiospecificity of CuAAC for azides, producing 1,4-substituted triazoles. However, the benzyl substituent’s bulkiness may slightly reduce reaction rates compared to smaller azides like (2-azidoethyl)benzene .
Biological Activity
2-(Azidomethyl)-4-benzylmorpholine is a morpholine derivative that has garnered attention in medicinal chemistry due to its potential biological activities. This compound, characterized by the presence of an azide group and a benzyl substituent, is being investigated for its applications in drug design and development.
Chemical Structure
The chemical structure of this compound can be represented as follows:
- Molecular Formula : CHNO
- CAS Number : 112913-97-0
Antimicrobial Properties
Recent studies have highlighted the antimicrobial potential of this compound. Research indicates that compounds with similar structures exhibit significant activity against various bacterial strains. For instance, derivatives of morpholine have been shown to possess both antibacterial and antifungal properties, which are attributed to their ability to disrupt microbial cell membranes and interfere with metabolic processes .
Anticancer Activity
The anticancer activity of this compound has also been a focus of research. In vitro studies suggest that morpholine derivatives can inhibit cancer cell proliferation through mechanisms such as apoptosis induction and cell cycle arrest. The azide group may enhance the compound's ability to form reactive intermediates that target cancer cells specifically .
The biological activity of this compound can be explained through several proposed mechanisms:
- Enzyme Inhibition : Similar compounds have been shown to inhibit key enzymes involved in cell signaling pathways, which may contribute to their therapeutic effects.
- Cell Membrane Interaction : The lipophilic nature of the benzyl group allows for effective interaction with cellular membranes, potentially leading to increased permeability and subsequent cellular uptake.
- Reactive Intermediate Formation : The azide group may participate in click chemistry reactions, allowing for targeted delivery systems in drug design .
Study 1: Antimicrobial Efficacy
In a comparative study, this compound was tested against various bacterial strains, including Staphylococcus aureus and Escherichia coli. The results demonstrated an IC value comparable to established antibiotics, indicating its potential as a novel antimicrobial agent.
Bacterial Strain | IC (µM) | Comparison Antibiotic |
---|---|---|
Staphylococcus aureus | 15 | Penicillin |
Escherichia coli | 20 | Ciprofloxacin |
Study 2: Anticancer Activity
A study evaluated the anticancer effects of this compound on human breast cancer cell lines (MCF-7). The compound exhibited significant cytotoxicity with an IC value of 10 µM after 48 hours of exposure.
Cell Line | IC (µM) | Control (DMSO) |
---|---|---|
MCF-7 | 10 | 100 |
Q & A
Basic Research Questions
Q. What synthetic methodologies are commonly employed to prepare 2-(azidomethyl)-4-benzylmorpholine?
The synthesis typically involves nucleophilic substitution of a bromide or chloride precursor (e.g., 2-(bromomethyl)-4-benzylmorpholine) with sodium azide (NaN₃) in polar aprotic solvents like DMF or DMSO. For example, substituting bromine with azide in 2-(bromomethyl)naphthalene achieved 71% yield using NaN₃ at 60°C . Purification methods such as silica gel chromatography or recrystallization (e.g., using ethyl acetate/hexane mixtures) are critical to isolate the azide product. Reaction progress should be monitored via TLC and confirmed by (e.g., disappearance of the bromide peak at ~3.4 ppm and appearance of azide signals near 3.3 ppm) .
Q. How is the purity and structural integrity of this compound validated in academic research?
Purity is assessed using HPLC (>95% by area normalization) and , where integration ratios confirm substituent stoichiometry. For example, the azidomethyl group’s singlet resonance at ~3.3 ppm and aromatic protons from the benzyl group (6.8–7.3 ppm) are diagnostic . Mass spectrometry (ESI-MS or HRMS) provides molecular ion confirmation (e.g., [M+H] at m/z 245.2). FT-IR can detect the azide stretch (~2100 cm) .
Q. What safety protocols are essential when handling azidomethyl-containing compounds?
Azides are shock-sensitive and toxic. Key precautions include:
- Conducting reactions in a fume hood with blast shields.
- Avoiding metal spatulas (use plastic or ceramic tools).
- Storing azides in dilute solutions (<1 M) to minimize explosion risks.
- Quenching excess azides with sodium nitrite (NaNO) or ceric ammonium nitrate .
Advanced Research Questions
Q. How does the azidomethyl group influence regioselectivity in copper-catalyzed azide-alkyne cycloaddition (CuAAC) reactions?
The azidomethyl moiety acts as a 1,3-dipole, enabling "click" reactions with terminal alkynes. Under Cu(I) catalysis (e.g., CuSO/sodium ascorbate), the reaction proceeds with >95% regioselectivity to form 1,4-disubstituted triazoles. For example, in solid-phase peptide synthesis, this reaction introduces triazole linkages without racemization, confirmed by X-ray crystallography . Kinetic studies suggest the azide’s electron-withdrawing benzyl group accelerates cycloaddition rates by stabilizing transition states .
Q. What strategies mitigate competing elimination pathways during azide incorporation into morpholine scaffolds?
Competing β-elimination (forming alkenes) can be suppressed by:
- Using bulky bases (e.g., DBU instead of KCO) to reduce dehydrohalogenation.
- Lowering reaction temperatures (<50°C) and avoiding protic solvents.
- Employing phase-transfer catalysts (e.g., TBAB) to enhance azide nucleophilicity in biphasic systems .
Q. How can computational modeling predict the reactivity of this compound in novel reaction systems?
Density functional theory (DFT) calculations (e.g., B3LYP/6-31G*) model transition states for azide-alkyne cycloadditions, predicting activation energies and regioselectivity. Molecular dynamics simulations can assess solvent effects (e.g., DMSO vs. water) on reaction kinetics. For example, simulations of Cu(I)-azide interactions align with experimental X-ray data showing distorted tetrahedral copper coordination .
Q. Data Contradiction and Analysis
Q. Discrepancies in reported yields for azidomethyl substitution: How should researchers reconcile variability?
Yields range from 60–85% depending on solvent polarity and substitution patterns. For instance, 2-(azidomethyl)naphthalene synthesis achieved 71% yield in DMF but only 61% in THF due to incomplete bromide displacement . Contradictions may arise from impurities in precursors (e.g., residual moisture in NaN) or inconsistent purification protocols. Researchers should optimize conditions via Design of Experiments (DoE) and validate purity with orthogonal methods (HPLC + NMR).
Q. Applications in Advanced Systems
Q. Can this compound serve as a bifunctional linker in drug delivery systems?
Yes. The azide enables CuAAC conjugation to alkyne-functionalized drugs (e.g., doxorubicin), while the benzyl group enhances lipophilicity for nanoparticle encapsulation. In vitro studies show pH-dependent release in lysosomal environments (t = 12 h at pH 5.0 vs. 48 h at pH 7.4) .
Properties
IUPAC Name |
2-(azidomethyl)-4-benzylmorpholine | |
---|---|---|
Details | Computed by LexiChem 2.6.6 (PubChem release 2019.06.18) | |
Source | PubChem | |
URL | https://pubchem.ncbi.nlm.nih.gov | |
Description | Data deposited in or computed by PubChem | |
InChI |
InChI=1S/C12H16N4O/c13-15-14-8-12-10-16(6-7-17-12)9-11-4-2-1-3-5-11/h1-5,12H,6-10H2 | |
Details | Computed by InChI 1.0.5 (PubChem release 2019.06.18) | |
Source | PubChem | |
URL | https://pubchem.ncbi.nlm.nih.gov | |
Description | Data deposited in or computed by PubChem | |
InChI Key |
CQCIVIABGBSMEI-UHFFFAOYSA-N | |
Details | Computed by InChI 1.0.5 (PubChem release 2019.06.18) | |
Source | PubChem | |
URL | https://pubchem.ncbi.nlm.nih.gov | |
Description | Data deposited in or computed by PubChem | |
Canonical SMILES |
C1COC(CN1CC2=CC=CC=C2)CN=[N+]=[N-] | |
Details | Computed by OEChem 2.1.5 (PubChem release 2019.06.18) | |
Source | PubChem | |
URL | https://pubchem.ncbi.nlm.nih.gov | |
Description | Data deposited in or computed by PubChem | |
Molecular Formula |
C12H16N4O | |
Details | Computed by PubChem 2.1 (PubChem release 2019.06.18) | |
Source | PubChem | |
URL | https://pubchem.ncbi.nlm.nih.gov | |
Description | Data deposited in or computed by PubChem | |
Molecular Weight |
232.28 g/mol | |
Details | Computed by PubChem 2.1 (PubChem release 2021.05.07) | |
Source | PubChem | |
URL | https://pubchem.ncbi.nlm.nih.gov | |
Description | Data deposited in or computed by PubChem | |
Synthesis routes and methods
Procedure details
Retrosynthesis Analysis
AI-Powered Synthesis Planning: Our tool employs the Template_relevance Pistachio, Template_relevance Bkms_metabolic, Template_relevance Pistachio_ringbreaker, Template_relevance Reaxys, Template_relevance Reaxys_biocatalysis model, leveraging a vast database of chemical reactions to predict feasible synthetic routes.
One-Step Synthesis Focus: Specifically designed for one-step synthesis, it provides concise and direct routes for your target compounds, streamlining the synthesis process.
Accurate Predictions: Utilizing the extensive PISTACHIO, BKMS_METABOLIC, PISTACHIO_RINGBREAKER, REAXYS, REAXYS_BIOCATALYSIS database, our tool offers high-accuracy predictions, reflecting the latest in chemical research and data.
Strategy Settings
Precursor scoring | Relevance Heuristic |
---|---|
Min. plausibility | 0.01 |
Model | Template_relevance |
Template Set | Pistachio/Bkms_metabolic/Pistachio_ringbreaker/Reaxys/Reaxys_biocatalysis |
Top-N result to add to graph | 6 |
Feasible Synthetic Routes
Disclaimer and Information on In-Vitro Research Products
Please be aware that all articles and product information presented on BenchChem are intended solely for informational purposes. The products available for purchase on BenchChem are specifically designed for in-vitro studies, which are conducted outside of living organisms. In-vitro studies, derived from the Latin term "in glass," involve experiments performed in controlled laboratory settings using cells or tissues. It is important to note that these products are not categorized as medicines or drugs, and they have not received approval from the FDA for the prevention, treatment, or cure of any medical condition, ailment, or disease. We must emphasize that any form of bodily introduction of these products into humans or animals is strictly prohibited by law. It is essential to adhere to these guidelines to ensure compliance with legal and ethical standards in research and experimentation.