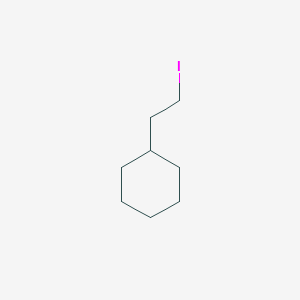
(2-Iodoethyl)cyclohexane
Overview
Description
(2-Iodoethyl)cyclohexane is an organoiodine compound with the molecular formula C8H15I It is a derivative of cyclohexane, where an ethyl group is substituted with an iodine atom at the second position
Preparation Methods
Synthetic Routes and Reaction Conditions
(2-Iodoethyl)cyclohexane can be synthesized through several methods. One common approach involves the addition of hydrogen iodide to cyclohexene, resulting in the formation of iodocyclohexane, which can then be further reacted to obtain this compound . Another method involves the reaction of cyclohexane with iodoform under specific conditions .
Industrial Production Methods
Industrial production of this compound typically involves large-scale synthesis using optimized reaction conditions to ensure high yield and purity. The process may include the use of catalysts and controlled environments to facilitate the reaction and minimize by-products.
Chemical Reactions Analysis
Types of Reactions
(2-Iodoethyl)cyclohexane undergoes various types of chemical reactions, including:
Substitution Reactions: The iodine atom can be substituted with other functional groups using appropriate reagents.
Oxidation and Reduction Reactions: The compound can undergo oxidation to form different products, depending on the reagents and conditions used.
Common Reagents and Conditions
Substitution Reactions: Common reagents include nucleophiles such as sodium azide or potassium cyanide.
Oxidation Reactions: Reagents like potassium permanganate or chromium trioxide can be used under acidic or basic conditions.
Major Products Formed
The major products formed from these reactions depend on the specific reagents and conditions used. For example, substitution reactions may yield compounds like (2-azidoethyl)cyclohexane or (2-cyanoethyl)cyclohexane.
Scientific Research Applications
(2-Iodoethyl)cyclohexane has several applications in scientific research:
Organic Synthesis: It is used as an intermediate in the synthesis of more complex organic molecules.
Medicinal Chemistry: The compound is explored for its potential use in the development of pharmaceuticals.
Material Science: It is used in the synthesis of materials with specific properties for industrial applications.
Mechanism of Action
The mechanism of action of (2-Iodoethyl)cyclohexane involves its interaction with various molecular targets. The iodine atom in the compound can participate in halogen bonding, influencing the reactivity and stability of the molecule. The pathways involved in its reactions depend on the specific conditions and reagents used.
Comparison with Similar Compounds
Similar Compounds
Iodocyclohexane: A similar compound where the iodine atom is directly attached to the cyclohexane ring.
Chlorocyclohexane: A compound where the iodine atom is replaced with a chlorine atom.
Bromocyclohexane: A compound where the iodine atom is replaced with a bromine atom.
Uniqueness
(2-Iodoethyl)cyclohexane is unique due to the presence of the ethyl group with an iodine atom, which imparts distinct chemical properties compared to other halogenated cyclohexanes. This uniqueness makes it valuable for specific applications in organic synthesis and medicinal chemistry.
Properties
IUPAC Name |
2-iodoethylcyclohexane | |
---|---|---|
Details | Computed by LexiChem 2.6.6 (PubChem release 2019.06.18) | |
Source | PubChem | |
URL | https://pubchem.ncbi.nlm.nih.gov | |
Description | Data deposited in or computed by PubChem | |
InChI |
InChI=1S/C8H15I/c9-7-6-8-4-2-1-3-5-8/h8H,1-7H2 | |
Details | Computed by InChI 1.0.5 (PubChem release 2019.06.18) | |
Source | PubChem | |
URL | https://pubchem.ncbi.nlm.nih.gov | |
Description | Data deposited in or computed by PubChem | |
InChI Key |
QQLSWNGYIUYOQV-UHFFFAOYSA-N | |
Details | Computed by InChI 1.0.5 (PubChem release 2019.06.18) | |
Source | PubChem | |
URL | https://pubchem.ncbi.nlm.nih.gov | |
Description | Data deposited in or computed by PubChem | |
Canonical SMILES |
C1CCC(CC1)CCI | |
Details | Computed by OEChem 2.1.5 (PubChem release 2019.06.18) | |
Source | PubChem | |
URL | https://pubchem.ncbi.nlm.nih.gov | |
Description | Data deposited in or computed by PubChem | |
Molecular Formula |
C8H15I | |
Details | Computed by PubChem 2.1 (PubChem release 2019.06.18) | |
Source | PubChem | |
URL | https://pubchem.ncbi.nlm.nih.gov | |
Description | Data deposited in or computed by PubChem | |
DSSTOX Substance ID |
DTXSID10513095 | |
Record name | (2-Iodoethyl)cyclohexane | |
Source | EPA DSSTox | |
URL | https://comptox.epa.gov/dashboard/DTXSID10513095 | |
Description | DSSTox provides a high quality public chemistry resource for supporting improved predictive toxicology. | |
Molecular Weight |
238.11 g/mol | |
Details | Computed by PubChem 2.1 (PubChem release 2021.05.07) | |
Source | PubChem | |
URL | https://pubchem.ncbi.nlm.nih.gov | |
Description | Data deposited in or computed by PubChem | |
CAS No. |
80203-35-6 | |
Record name | (2-Iodoethyl)cyclohexane | |
Source | EPA DSSTox | |
URL | https://comptox.epa.gov/dashboard/DTXSID10513095 | |
Description | DSSTox provides a high quality public chemistry resource for supporting improved predictive toxicology. | |
Synthesis routes and methods I
Procedure details
Synthesis routes and methods II
Procedure details
Retrosynthesis Analysis
AI-Powered Synthesis Planning: Our tool employs the Template_relevance Pistachio, Template_relevance Bkms_metabolic, Template_relevance Pistachio_ringbreaker, Template_relevance Reaxys, Template_relevance Reaxys_biocatalysis model, leveraging a vast database of chemical reactions to predict feasible synthetic routes.
One-Step Synthesis Focus: Specifically designed for one-step synthesis, it provides concise and direct routes for your target compounds, streamlining the synthesis process.
Accurate Predictions: Utilizing the extensive PISTACHIO, BKMS_METABOLIC, PISTACHIO_RINGBREAKER, REAXYS, REAXYS_BIOCATALYSIS database, our tool offers high-accuracy predictions, reflecting the latest in chemical research and data.
Strategy Settings
Precursor scoring | Relevance Heuristic |
---|---|
Min. plausibility | 0.01 |
Model | Template_relevance |
Template Set | Pistachio/Bkms_metabolic/Pistachio_ringbreaker/Reaxys/Reaxys_biocatalysis |
Top-N result to add to graph | 6 |
Feasible Synthetic Routes
Disclaimer and Information on In-Vitro Research Products
Please be aware that all articles and product information presented on BenchChem are intended solely for informational purposes. The products available for purchase on BenchChem are specifically designed for in-vitro studies, which are conducted outside of living organisms. In-vitro studies, derived from the Latin term "in glass," involve experiments performed in controlled laboratory settings using cells or tissues. It is important to note that these products are not categorized as medicines or drugs, and they have not received approval from the FDA for the prevention, treatment, or cure of any medical condition, ailment, or disease. We must emphasize that any form of bodily introduction of these products into humans or animals is strictly prohibited by law. It is essential to adhere to these guidelines to ensure compliance with legal and ethical standards in research and experimentation.