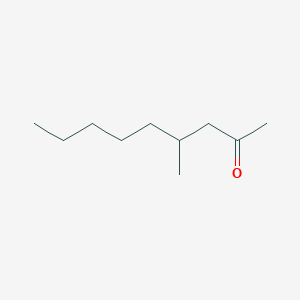
4-Methyl-2-nonanone
Overview
Description
4-Methyl-2-nonanone: is an organic compound with the chemical formula C10H20O . It is a type of methyl ketone, characterized by a ketone functional group attached to a nonane backbone. This compound is known for its use in various industrial applications due to its unique chemical properties .
Preparation Methods
Synthetic Routes and Reaction Conditions: 4-Methyl-2-nonanone can be synthesized through several methods. One common method involves the oxidation of 4-methyl-2-nonanol using an oxidizing agent such as potassium permanganate or chromium trioxide. Another method involves the condensation of acetone with butyraldehyde, followed by hydrogenation .
Industrial Production Methods: In industrial settings, this compound is often produced through the catalytic hydrogenation of 4-methyl-2-nonanol. This process involves the use of a metal catalyst, such as palladium or platinum, under high pressure and temperature conditions .
Chemical Reactions Analysis
Types of Reactions: 4-Methyl-2-nonanone undergoes various chemical reactions, including:
Oxidation: It can be oxidized to form carboxylic acids using strong oxidizing agents.
Reduction: It can be reduced to form alcohols using reducing agents like lithium aluminum hydride or sodium borohydride.
Substitution: It can undergo nucleophilic substitution reactions, where the ketone group is replaced by other functional groups.
Common Reagents and Conditions:
Oxidation: Potassium permanganate, chromium trioxide.
Reduction: Lithium aluminum hydride, sodium borohydride.
Substitution: Various nucleophiles under acidic or basic conditions.
Major Products:
Oxidation: Carboxylic acids.
Reduction: Alcohols.
Substitution: Compounds with different functional groups replacing the ketone group.
Scientific Research Applications
4-Methyl-2-nonanone has several applications in scientific research:
Chemistry: It is used as a solvent and intermediate in organic synthesis.
Biology: It is studied for its potential effects on biological systems, including its role as a pheromone in certain insect species.
Medicine: Research is ongoing to explore its potential therapeutic applications.
Industry: It is used in the production of fragrances, flavors, and other chemical products.
Mechanism of Action
The mechanism of action of 4-Methyl-2-nonanone involves its interaction with various molecular targets. In biological systems, it can act as a pheromone, influencing the behavior of certain insects. The exact molecular pathways involved in its action are still under investigation, but it is believed to interact with specific receptors and enzymes .
Comparison with Similar Compounds
2-Nonanone: Similar in structure but lacks the methyl group at the fourth position.
4-Methyl-2-nonanol: The alcohol counterpart of 4-Methyl-2-nonanone.
2-Heptanone: A shorter chain methyl ketone with similar chemical properties.
Uniqueness: this compound is unique due to its specific structure, which imparts distinct chemical and physical properties. Its methyl group at the fourth position and the ketone functional group at the second position make it particularly useful in various industrial and research applications .
Properties
IUPAC Name |
4-methylnonan-2-one | |
---|---|---|
Details | Computed by LexiChem 2.6.6 (PubChem release 2019.06.18) | |
Source | PubChem | |
URL | https://pubchem.ncbi.nlm.nih.gov | |
Description | Data deposited in or computed by PubChem | |
InChI |
InChI=1S/C10H20O/c1-4-5-6-7-9(2)8-10(3)11/h9H,4-8H2,1-3H3 | |
Details | Computed by InChI 1.0.5 (PubChem release 2019.06.18) | |
Source | PubChem | |
URL | https://pubchem.ncbi.nlm.nih.gov | |
Description | Data deposited in or computed by PubChem | |
InChI Key |
JBYKHOKWOUCUDJ-UHFFFAOYSA-N | |
Details | Computed by InChI 1.0.5 (PubChem release 2019.06.18) | |
Source | PubChem | |
URL | https://pubchem.ncbi.nlm.nih.gov | |
Description | Data deposited in or computed by PubChem | |
Canonical SMILES |
CCCCCC(C)CC(=O)C | |
Details | Computed by OEChem 2.1.5 (PubChem release 2019.06.18) | |
Source | PubChem | |
URL | https://pubchem.ncbi.nlm.nih.gov | |
Description | Data deposited in or computed by PubChem | |
Molecular Formula |
C10H20O | |
Details | Computed by PubChem 2.1 (PubChem release 2019.06.18) | |
Source | PubChem | |
URL | https://pubchem.ncbi.nlm.nih.gov | |
Description | Data deposited in or computed by PubChem | |
Molecular Weight |
156.26 g/mol | |
Details | Computed by PubChem 2.1 (PubChem release 2021.05.07) | |
Source | PubChem | |
URL | https://pubchem.ncbi.nlm.nih.gov | |
Description | Data deposited in or computed by PubChem | |
Retrosynthesis Analysis
AI-Powered Synthesis Planning: Our tool employs the Template_relevance Pistachio, Template_relevance Bkms_metabolic, Template_relevance Pistachio_ringbreaker, Template_relevance Reaxys, Template_relevance Reaxys_biocatalysis model, leveraging a vast database of chemical reactions to predict feasible synthetic routes.
One-Step Synthesis Focus: Specifically designed for one-step synthesis, it provides concise and direct routes for your target compounds, streamlining the synthesis process.
Accurate Predictions: Utilizing the extensive PISTACHIO, BKMS_METABOLIC, PISTACHIO_RINGBREAKER, REAXYS, REAXYS_BIOCATALYSIS database, our tool offers high-accuracy predictions, reflecting the latest in chemical research and data.
Strategy Settings
Precursor scoring | Relevance Heuristic |
---|---|
Min. plausibility | 0.01 |
Model | Template_relevance |
Template Set | Pistachio/Bkms_metabolic/Pistachio_ringbreaker/Reaxys/Reaxys_biocatalysis |
Top-N result to add to graph | 6 |
Feasible Synthetic Routes
Disclaimer and Information on In-Vitro Research Products
Please be aware that all articles and product information presented on BenchChem are intended solely for informational purposes. The products available for purchase on BenchChem are specifically designed for in-vitro studies, which are conducted outside of living organisms. In-vitro studies, derived from the Latin term "in glass," involve experiments performed in controlled laboratory settings using cells or tissues. It is important to note that these products are not categorized as medicines or drugs, and they have not received approval from the FDA for the prevention, treatment, or cure of any medical condition, ailment, or disease. We must emphasize that any form of bodily introduction of these products into humans or animals is strictly prohibited by law. It is essential to adhere to these guidelines to ensure compliance with legal and ethical standards in research and experimentation.