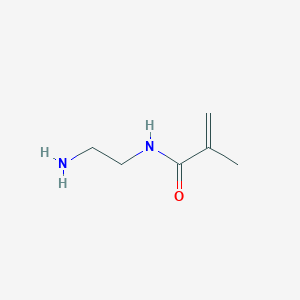
N'-methacryloyl-ethylenediamine
Overview
Description
N’-methacryloyl-ethylenediamine is a chemical compound that contains a total of 21 atoms; 12 Hydrogen atoms, 6 Carbon atoms, 2 Nitrogen atoms, and 1 Oxygen atom . It is used as a cross-linker in the synthesis of novel magnetic molecularly imprinted polymers (MMIPs) .
Synthesis Analysis
The MMIPs were synthesized using N, N -bis methacryloyl ethylenediamine as a cross-linker for the controlled release of meloxicam at different pH levels . The MMIPs were prepared via precipitation polymerization, using Fe3O4 as a magnetic component, meloxicam as a template molecule, methacrylic acid (MAA) as a functional monomer, and N, N -bis methacryloyl ethylenediamine as a new cross-linker in acetonitrile/dimethyl sulfoxide porogen .Molecular Structure Analysis
The molecular structure of N’-methacryloyl-ethylenediamine consists of 12 Hydrogen atoms, 6 Carbon atoms, 2 Nitrogen atoms, and 1 Oxygen atom .Chemical Reactions Analysis
The chemical reaction involving N’-methacryloyl-ethylenediamine in the synthesis of MMIPs proceeds through a sequential acyl radical addition/cyclization strategy .Mechanism of Action
The mechanism of action of N’-methacryloyl-ethylenediamine in the synthesis of MMIPs involves the formation of binding sites that have affinity for a particular template (or target) molecule . These sites are created through interactions between the template and selected monomer units possessing complementary functional groups that form a cavity within the gross polymer .
Safety and Hazards
Future Directions
The results indicated that the magnetic MIPs synthesized using N, N -bis methacryloyl ethylenediamine as a cross-linker also had potential applications in drug controlled release . This suggests that N’-methacryloyl-ethylenediamine could play a significant role in the development of new drug delivery systems in the future.
properties
CAS RN |
63298-57-7 |
---|---|
Product Name |
N'-methacryloyl-ethylenediamine |
Molecular Formula |
C6H12N2O |
Molecular Weight |
128.17 g/mol |
IUPAC Name |
N-(2-aminoethyl)-2-methylprop-2-enamide |
InChI |
InChI=1S/C6H12N2O/c1-5(2)6(9)8-4-3-7/h1,3-4,7H2,2H3,(H,8,9) |
InChI Key |
RFZRLVGQBIINKQ-UHFFFAOYSA-N |
SMILES |
CC(=C)C(=O)NCCN |
Canonical SMILES |
CC(=C)C(=O)NCCN |
Origin of Product |
United States |
Synthesis routes and methods
Procedure details
Disclaimer and Information on In-Vitro Research Products
Please be aware that all articles and product information presented on BenchChem are intended solely for informational purposes. The products available for purchase on BenchChem are specifically designed for in-vitro studies, which are conducted outside of living organisms. In-vitro studies, derived from the Latin term "in glass," involve experiments performed in controlled laboratory settings using cells or tissues. It is important to note that these products are not categorized as medicines or drugs, and they have not received approval from the FDA for the prevention, treatment, or cure of any medical condition, ailment, or disease. We must emphasize that any form of bodily introduction of these products into humans or animals is strictly prohibited by law. It is essential to adhere to these guidelines to ensure compliance with legal and ethical standards in research and experimentation.