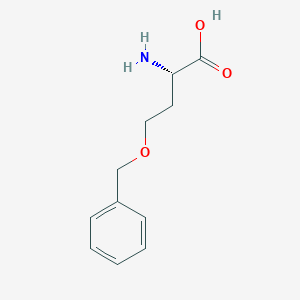
O-Benzyl-L-homoserine
Overview
Description
O-Benzyl-L-homoserine: is a synthetic analog of the naturally occurring amino acid homoserine. It is characterized by the presence of a benzyl group attached to the oxygen atom of the homoserine molecule. The molecular formula of this compound is C11H15NO3, and it has a molecular weight of 209.24 g/mol
Mechanism of Action
Target of Action
O-Benzyl-L-homoserine is a derivative of L-homoserine, which is an intermediate in the biosynthesis of three essential amino acids: methionine, threonine, and isoleucine . The primary targets of this compound are likely to be similar to those of L-homoserine. For instance, it has been found that L-homoserine allosterically inhibits aspartate kinase and glutamate dehydrogenase .
Mode of Action
It is known that l-homoserine, the parent compound, is converted to o-succinyl homoserine by homoserine o-succinyltransferase, a precursor to l-methionine . It is plausible that this compound might interact with its targets in a similar manner, leading to changes in the metabolic pathways involved in the synthesis of these amino acids.
Biochemical Pathways
This compound likely affects the same biochemical pathways as L-homoserine. L-homoserine is an intermediate in the biosynthesis of methionine, threonine, and isoleucine . Its complete biosynthetic pathway includes glycolysis, the tricarboxylic acid (TCA) or citric acid cycle (Krebs cycle), and the aspartate metabolic pathway .
Pharmacokinetics
It is known that the compound is stable at room temperature , which suggests that it may have good bioavailability
Biochemical Analysis
Biochemical Properties
O-Benzyl-L-homoserine is involved in the synthesis of homocysteine with the participation of an inorganic sulfur source directly from O-acetylhomoserine, which is predominant in most classes of bacteria . The enzyme homoserine dehydrogenase, in association with NADPH, catalyzes a reversible reaction that interconverts L-aspartate-4-semialdehyde to L-homoserine . Then, two other enzymes, homoserine kinase and homoserine O-succinyltransferase use homoserine as a substrate and produce phosphohomoserine and O-succinyl homoserine respectively .
Cellular Effects
This compound has been shown to influence cell function, particularly in relation to cell signaling pathways, gene expression, and cellular metabolism . For instance, in Escherichia coli, it has been found that overexpression of glycerol 3-phosphate dehydrogenase improved the OAH production .
Molecular Mechanism
The molecular mechanism of this compound involves its interaction with various biomolecules. Specifically, the enzyme homoserine dehydrogenase, in association with NADPH, catalyzes a reversible reaction that interconverts L-aspartate-4-semialdehyde to L-homoserine . This process involves binding interactions with biomolecules, enzyme inhibition or activation, and changes in gene expression.
Temporal Effects in Laboratory Settings
In laboratory settings, the effects of this compound can change over time. For instance, in a study involving Escherichia coli, it was found that the OAH titer increased 2.3-fold to 3.2 g/L when various feedstocks were added . This indicates that the product’s stability, degradation, and long-term effects on cellular function can be observed in in vitro or in vivo studies.
Metabolic Pathways
This compound is involved in the biosynthesis of three essential amino acids: methionine, threonine, and isoleucine . Its complete biosynthetic pathway includes glycolysis, the tricarboxylic acid (TCA) or citric acid cycle (Krebs cycle), and the aspartate metabolic pathway .
Subcellular Localization
The subcellular localization of this compound is not explicitly known. Given its role in various biochemical reactions, it is likely that it is localized in the cytoplasm where these reactions typically occur . This could include any targeting signals or post-translational modifications that direct it to specific compartments or organelles.
Preparation Methods
Synthetic Routes and Reaction Conditions: O-Benzyl-L-homoserine can be synthesized through several methods. One common approach involves the protection of the hydroxyl group of homoserine with a benzyl group. This can be achieved by reacting homoserine with benzyl chloride in the presence of a base such as sodium hydroxide. The reaction typically occurs under mild conditions and results in the formation of this compound .
Industrial Production Methods: Industrial production of this compound may involve large-scale synthesis using similar methods as described above. The process can be optimized for higher yields and purity by controlling reaction parameters such as temperature, pH, and reaction time. Additionally, purification techniques such as recrystallization or chromatography may be employed to obtain the desired product.
Chemical Reactions Analysis
Types of Reactions: O-Benzyl-L-homoserine undergoes various chemical reactions, including:
Oxidation: The compound can be oxidized to form corresponding oxo derivatives.
Reduction: Reduction reactions can convert this compound to its reduced forms.
Substitution: The benzyl group can be substituted with other functional groups under appropriate conditions.
Common Reagents and Conditions:
Oxidation: Common oxidizing agents such as potassium permanganate or chromium trioxide can be used.
Reduction: Reducing agents like lithium aluminum hydride or sodium borohydride are typically employed.
Substitution: Substitution reactions may involve reagents such as halogens or nucleophiles under specific conditions.
Major Products Formed:
Oxidation: Oxo derivatives of this compound.
Reduction: Reduced forms of the compound.
Substitution: Various substituted derivatives depending on the reagents used.
Scientific Research Applications
Chemistry: O-Benzyl-L-homoserine is used as a building block in organic synthesis. It serves as a precursor for the synthesis of more complex molecules and can be utilized in the development of new chemical reactions and methodologies .
Biology: In biological research, this compound is studied for its potential role in metabolic pathways. It can be used to investigate the biosynthesis of amino acids and other related compounds .
Medicine: It can be used as a starting material for the synthesis of pharmaceutical agents and may exhibit biological activity that could be harnessed for therapeutic purposes .
Industry: this compound is used in the production of fine chemicals and specialty materials. Its unique chemical properties make it valuable in various industrial processes .
Comparison with Similar Compounds
O-Acetyl-L-homoserine: Another derivative of homoserine with an acetyl group instead of a benzyl group.
O-Succinyl-L-homoserine: A homoserine derivative with a succinyl group.
O-Ethyl-L-homoserine: A homoserine derivative with an ethyl group.
Uniqueness: O-Benzyl-L-homoserine is unique due to the presence of the benzyl group, which imparts distinct chemical and physical properties. This makes it different from other homoserine derivatives and allows for specific applications in research and industry .
Properties
IUPAC Name |
(2S)-2-amino-4-phenylmethoxybutanoic acid | |
---|---|---|
Details | Computed by LexiChem 2.6.6 (PubChem release 2019.06.18) | |
Source | PubChem | |
URL | https://pubchem.ncbi.nlm.nih.gov | |
Description | Data deposited in or computed by PubChem | |
InChI |
InChI=1S/C11H15NO3/c12-10(11(13)14)6-7-15-8-9-4-2-1-3-5-9/h1-5,10H,6-8,12H2,(H,13,14)/t10-/m0/s1 | |
Details | Computed by InChI 1.0.5 (PubChem release 2019.06.18) | |
Source | PubChem | |
URL | https://pubchem.ncbi.nlm.nih.gov | |
Description | Data deposited in or computed by PubChem | |
InChI Key |
QTPSXPIAJFBGLO-JTQLQIEISA-N | |
Details | Computed by InChI 1.0.5 (PubChem release 2019.06.18) | |
Source | PubChem | |
URL | https://pubchem.ncbi.nlm.nih.gov | |
Description | Data deposited in or computed by PubChem | |
Canonical SMILES |
C1=CC=C(C=C1)COCCC(C(=O)O)N | |
Details | Computed by OEChem 2.1.5 (PubChem release 2019.06.18) | |
Source | PubChem | |
URL | https://pubchem.ncbi.nlm.nih.gov | |
Description | Data deposited in or computed by PubChem | |
Isomeric SMILES |
C1=CC=C(C=C1)COCC[C@@H](C(=O)O)N | |
Details | Computed by OEChem 2.1.5 (PubChem release 2019.06.18) | |
Source | PubChem | |
URL | https://pubchem.ncbi.nlm.nih.gov | |
Description | Data deposited in or computed by PubChem | |
Molecular Formula |
C11H15NO3 | |
Details | Computed by PubChem 2.1 (PubChem release 2019.06.18) | |
Source | PubChem | |
URL | https://pubchem.ncbi.nlm.nih.gov | |
Description | Data deposited in or computed by PubChem | |
DSSTOX Substance ID |
DTXSID80679814 | |
Record name | O-Benzyl-L-homoserine | |
Source | EPA DSSTox | |
URL | https://comptox.epa.gov/dashboard/DTXSID80679814 | |
Description | DSSTox provides a high quality public chemistry resource for supporting improved predictive toxicology. | |
Molecular Weight |
209.24 g/mol | |
Details | Computed by PubChem 2.1 (PubChem release 2021.05.07) | |
Source | PubChem | |
URL | https://pubchem.ncbi.nlm.nih.gov | |
Description | Data deposited in or computed by PubChem | |
CAS No. |
62965-20-2 | |
Record name | O-Benzyl-L-homoserine | |
Source | EPA DSSTox | |
URL | https://comptox.epa.gov/dashboard/DTXSID80679814 | |
Description | DSSTox provides a high quality public chemistry resource for supporting improved predictive toxicology. | |
Retrosynthesis Analysis
AI-Powered Synthesis Planning: Our tool employs the Template_relevance Pistachio, Template_relevance Bkms_metabolic, Template_relevance Pistachio_ringbreaker, Template_relevance Reaxys, Template_relevance Reaxys_biocatalysis model, leveraging a vast database of chemical reactions to predict feasible synthetic routes.
One-Step Synthesis Focus: Specifically designed for one-step synthesis, it provides concise and direct routes for your target compounds, streamlining the synthesis process.
Accurate Predictions: Utilizing the extensive PISTACHIO, BKMS_METABOLIC, PISTACHIO_RINGBREAKER, REAXYS, REAXYS_BIOCATALYSIS database, our tool offers high-accuracy predictions, reflecting the latest in chemical research and data.
Strategy Settings
Precursor scoring | Relevance Heuristic |
---|---|
Min. plausibility | 0.01 |
Model | Template_relevance |
Template Set | Pistachio/Bkms_metabolic/Pistachio_ringbreaker/Reaxys/Reaxys_biocatalysis |
Top-N result to add to graph | 6 |
Feasible Synthetic Routes
Disclaimer and Information on In-Vitro Research Products
Please be aware that all articles and product information presented on BenchChem are intended solely for informational purposes. The products available for purchase on BenchChem are specifically designed for in-vitro studies, which are conducted outside of living organisms. In-vitro studies, derived from the Latin term "in glass," involve experiments performed in controlled laboratory settings using cells or tissues. It is important to note that these products are not categorized as medicines or drugs, and they have not received approval from the FDA for the prevention, treatment, or cure of any medical condition, ailment, or disease. We must emphasize that any form of bodily introduction of these products into humans or animals is strictly prohibited by law. It is essential to adhere to these guidelines to ensure compliance with legal and ethical standards in research and experimentation.