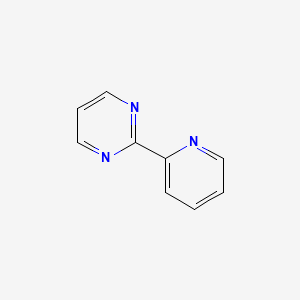
2-(Pyridin-2-yl)pyrimidine
Description
2-(Pyridin-2-yl)pyrimidine is a heterocyclic compound featuring a pyrimidine ring directly bonded to a pyridine ring at the 2-position. This structure serves as a versatile scaffold in medicinal chemistry due to its ability to engage in hydrogen bonding and π-π stacking interactions, which are critical for binding biological targets. Recent studies have focused on its derivatives as inhibitors of collagen prolyl-4-hydroxylases (CP4Hs), enzymes implicated in fibrosis by promoting collagen deposition .
The synthesis of these derivatives typically involves multi-step routes, such as esterification, oxidation, nucleophilic substitution, and cyclization reactions. For example, ethyl 6-aminonicotinate is oxidized to a pyridine N-oxide intermediate, followed by cyanide substitution and cyclization to yield the core this compound structure . Key intermediates like compound 5 (ethyl 6-cyanonicotinate) are critical for further functionalization with aryl or alkyl substituents, enhancing target specificity and potency .
Q & A
Q. What are the common synthetic routes for 2-(Pyridin-2-yl)pyrimidine derivatives, and how are reaction conditions optimized for high yield?
Basic
The synthesis of this compound derivatives typically involves multi-step reactions such as nucleophilic substitution, Suzuki-Miyaura coupling, or acid-catalyzed cyclization. For example, derivatives like 4-(((4-Chlorobenzyl)sulfanyl)methyl)-6-((2,4-dichlorobenzyl)sulfanyl)-2-(2-pyridinyl)pyrimidine are synthesized via sequential thiol substitution and coupling reactions using chlorobenzyl groups and pyridinylpyrimidine cores . Key parameters for optimization include:
- Temperature : Elevated temperatures (80–120°C) enhance reaction rates but require careful control to avoid side reactions.
- Catalysts : Palladium catalysts (e.g., Pd(PPh₃)₄) improve coupling efficiency in cross-coupling reactions.
- Purification : Column chromatography or recrystallization ensures >95% purity .
Q. How can researchers resolve contradictions in biological activity data across different cell lines for this compound derivatives?
Advanced
Discrepancies in biological activity (e.g., varying IC₅₀ values in K562 vs. HO-8910 cancer cells) often arise from differences in cell permeability, metabolic stability, or target expression. Methodological approaches include:
- Mechanistic Profiling : Use kinase inhibition assays or proteomics to identify off-target effects .
- Pharmacokinetic Studies : Assess metabolic stability in liver microsomes to correlate in vitro and in vivo activity .
- Structure-Activity Relationship (SAR) Analysis : Modify substituents (e.g., sulfonyl or trifluoromethyl groups) to enhance selectivity .
Q. What spectroscopic and crystallographic techniques are critical for characterizing this compound derivatives?
Basic
- Nuclear Magnetic Resonance (NMR) : ¹H and ¹³C NMR confirm regiochemistry and substituent positions (e.g., δ 8.2–8.5 ppm for pyridinyl protons) .
- Mass Spectrometry (MS) : High-resolution ESI-MS validates molecular weights (e.g., [M+H]⁺ at m/z 456.12 for a derivative) .
- X-ray Crystallography : SHELX software refines crystal structures to resolve bond lengths and angles (e.g., C–N bond distances of 1.33–1.37 Å) .
Q. How can computational methods predict the binding modes of this compound derivatives to enzyme targets?
Advanced
- Molecular Docking : Tools like AutoDock Vina simulate interactions with active sites (e.g., methionine aminopeptidase-1) using PyMOL for visualization .
- Density Functional Theory (DFT) : Calculates electrostatic potential maps to identify nucleophilic/electrophilic regions on the pyrimidine ring .
- MD Simulations : Assess binding stability over 100-ns trajectories to prioritize derivatives for synthesis .
Q. What strategies improve selectivity in enzyme inhibition for pyridinylpyrimidine-based compounds?
Advanced
- Fragment-Based Design : Introduce bulky substituents (e.g., tert-butyl groups) to block non-target binding pockets .
- Isosteric Replacement : Replace oxygen with sulfur in sulfonyl groups to modulate hydrogen bonding .
- Co-crystallization Studies : Resolve target-ligand complexes (e.g., with SHELX-refined structures) to guide modifications .
Q. How should researchers handle crystallographic disorder in X-ray structures of pyridinylpyrimidine derivatives?
Advanced
- Multi-Refinement Cycles : Use SHELXL to refine occupancy ratios for disordered atoms (e.g., solvent molecules in lattice voids) .
- Twinned Data Correction : Apply HKL-3000 or CrysAlis PRO to deconvolute overlapping reflections .
- Temperature Factor Analysis : B-factor thresholds (< 80 Ų) ensure reliable atomic displacement parameters .
Q. What are the key steps in multi-step synthesis of this compound derivatives with heterocyclic fused rings?
Basic
Core Formation : Condense pyridine-2-carboxaldehyde with thiourea under acidic conditions to form the pyrimidine ring .
Functionalization : Introduce substituents via Pd-catalyzed cross-coupling or nucleophilic aromatic substitution .
Purification : Sequential column chromatography (silica gel, eluent: EtOAc/hexane) isolates intermediates .
Q. How can stability studies assess the shelf-life of this compound derivatives under varying pH and temperature?
Advanced
- Forced Degradation : Expose compounds to 0.1M HCl/NaOH (70°C, 24h) and analyze degradation products via LC-MS .
- Accelerated Aging : Store samples at 40°C/75% RH for 6 months; monitor purity by HPLC .
- Light Sensitivity : UV-Vis spectroscopy tracks photodegradation (λmax 260–280 nm) under ICH Q1B guidelines .
Q. What experimental designs validate target engagement of pyridinylpyrimidine derivatives in cellular models?
Advanced
- Cellular Thermal Shift Assay (CETSA) : Monitor thermal stabilization of target proteins (e.g., kinases) after compound treatment .
- RNA Interference (RNAi) : Knock down putative targets (e.g., DNMT1) and compare compound efficacy in siRNA-treated vs. control cells .
- BRET/FRET Assays : Quantify real-time target-ligand interactions in live cells .
Q. How can researchers address low solubility of pyridinylpyrimidine derivatives in biological assays?
Advanced
- Salt Formation : Convert free bases to hydrochloride salts (e.g., this compound dihydrochloride) .
- Nanoparticle Formulation : Encapsulate compounds in PLGA nanoparticles (size: 100–200 nm) to enhance bioavailability .
- Co-solvent Systems : Use DMSO/PEG-400 mixtures (<5% v/v) to maintain solubility without cytotoxicity .
Comparison with Similar Compounds
Structural and Functional Modifications
The anti-fibrotic activity of 2-(pyridin-2-yl)pyrimidine derivatives is highly dependent on substituent groups at the pyrimidine and pyridine rings. Below is a comparative analysis based on structural variations and their biological effects:
Substituent Group R (Ethyl vs. Hydrogen)
Ethyl-substituted derivatives (e.g., 12m , 12q ) consistently outperform hydrogen-substituted analogs (e.g., 13m , 13q ) in inhibiting hepatic stellate cell (HSC-T6) proliferation. For instance:
- 12m (ethyl 6-(5-(p-tolylcarbamoyl)pyrimidin-2-yl)nicotinate): IC50 = 45.69 µM.
- 13m (6-(5-(p-tolylcarbamoyl)pyrimidin-2-yl)nicotinic acid): IC50 > 1000 µM .
The ethyl group likely enhances lipophilicity, improving membrane permeability and target engagement.
Substituent Position on Aromatic Rings
- 4-Substituted Phenyl Derivatives : Compounds like 12l (4-bromophenyl) and 12m (4-methylphenyl) exhibit superior activity (IC50 ~45–60 µM) compared to 3-substituted analogs such as 12n (3-bromophenyl, IC50 = 259 µM) .
- Electron-Withdrawing Groups : Fluorine or chlorine at the para position (12k , 4-chlorophenyl; 12q , 3,4-difluorophenyl) enhances inhibitory effects, likely due to increased electrophilicity and binding affinity .
Phenyl vs. Benzyl Substituents
Derivatives with phenyl rings directly attached to the pyrimidine core (e.g., 12k–12q ) show stronger anti-fibrotic activity than benzyl-substituted analogs (e.g., 12b , 12c ). This suggests steric hindrance from the benzyl group reduces target accessibility .
Activity Data of Key Derivatives
Compound | Substituent (R1) | IC50 (µM) | Molecular Weight | Key Feature(s) |
---|---|---|---|---|
12m | 4-Methylphenyl | 45.69 | 363.37 | Ethyl ester, high lipophilicity |
12q | 3,4-Difluorophenyl | 45.81 | 385.35 | Dual fluorine, enhanced binding |
12l | 4-Bromophenyl | 60.00 | 427.34 | Bromine, bulky substituent |
13m | 4-Methylphenyl (H-subst.) | >1000 | 355.39 | Carboxylic acid, reduced activity |
Pirfenidone | — | 250–300 | 185.18 | Reference drug, moderate activity |
Mechanistic Insights
The most active derivatives, 12m and 12q , suppress collagen type Iα1 (COL1A1) expression and hydroxyproline content in HSC-T6 cells by >50%, outperforming Pirfenidone . Their efficacy is attributed to:
Inhibition of TGF-β1 Signaling : Downregulation of TGF-β1 mRNA, a key driver of fibrogenesis .
CP4H Binding : Direct interaction with CP4H active sites, disrupting collagen maturation .
Physicochemical and Spectroscopic Properties
- Solubility : Ethyl esters (12m , 12q ) exhibit better solubility in organic solvents (e.g., DCM, EtOAc) than carboxylic acid derivatives (13m , 13q ), as confirmed by NMR spectra in DMSO-d6 .
- Thermal Stability : Melting points range from 189°C (12s ) to 312°C (13p ), with higher stability observed in halogenated derivatives .
- Mass Spectrometry : Molecular ions ([M+H]+) align with theoretical values (e.g., 12m : 363.37 vs. observed 363.35 ).
Properties
IUPAC Name |
2-pyridin-2-ylpyrimidine | |
---|---|---|
Details | Computed by Lexichem TK 2.7.0 (PubChem release 2021.10.14) | |
Source | PubChem | |
URL | https://pubchem.ncbi.nlm.nih.gov | |
Description | Data deposited in or computed by PubChem | |
InChI |
InChI=1S/C9H7N3/c1-2-5-10-8(4-1)9-11-6-3-7-12-9/h1-7H | |
Details | Computed by InChI 1.0.6 (PubChem release 2021.10.14) | |
Source | PubChem | |
URL | https://pubchem.ncbi.nlm.nih.gov | |
Description | Data deposited in or computed by PubChem | |
InChI Key |
YJVKLLJCUMQBHN-UHFFFAOYSA-N | |
Details | Computed by InChI 1.0.6 (PubChem release 2021.10.14) | |
Source | PubChem | |
URL | https://pubchem.ncbi.nlm.nih.gov | |
Description | Data deposited in or computed by PubChem | |
Canonical SMILES |
C1=CC=NC(=C1)C2=NC=CC=N2 | |
Details | Computed by OEChem 2.3.0 (PubChem release 2021.10.14) | |
Source | PubChem | |
URL | https://pubchem.ncbi.nlm.nih.gov | |
Description | Data deposited in or computed by PubChem | |
Molecular Formula |
C9H7N3 | |
Details | Computed by PubChem 2.2 (PubChem release 2021.10.14) | |
Source | PubChem | |
URL | https://pubchem.ncbi.nlm.nih.gov | |
Description | Data deposited in or computed by PubChem | |
DSSTOX Substance ID |
DTXSID10437969 | |
Record name | Pyrimidine, 2-(2-pyridinyl)- | |
Source | EPA DSSTox | |
URL | https://comptox.epa.gov/dashboard/DTXSID10437969 | |
Description | DSSTox provides a high quality public chemistry resource for supporting improved predictive toxicology. | |
Molecular Weight |
157.17 g/mol | |
Details | Computed by PubChem 2.2 (PubChem release 2021.10.14) | |
Source | PubChem | |
URL | https://pubchem.ncbi.nlm.nih.gov | |
Description | Data deposited in or computed by PubChem | |
CAS No. |
10198-83-1 | |
Record name | Pyrimidine, 2-(2-pyridinyl)- | |
Source | EPA DSSTox | |
URL | https://comptox.epa.gov/dashboard/DTXSID10437969 | |
Description | DSSTox provides a high quality public chemistry resource for supporting improved predictive toxicology. | |
Disclaimer and Information on In-Vitro Research Products
Please be aware that all articles and product information presented on BenchChem are intended solely for informational purposes. The products available for purchase on BenchChem are specifically designed for in-vitro studies, which are conducted outside of living organisms. In-vitro studies, derived from the Latin term "in glass," involve experiments performed in controlled laboratory settings using cells or tissues. It is important to note that these products are not categorized as medicines or drugs, and they have not received approval from the FDA for the prevention, treatment, or cure of any medical condition, ailment, or disease. We must emphasize that any form of bodily introduction of these products into humans or animals is strictly prohibited by law. It is essential to adhere to these guidelines to ensure compliance with legal and ethical standards in research and experimentation.