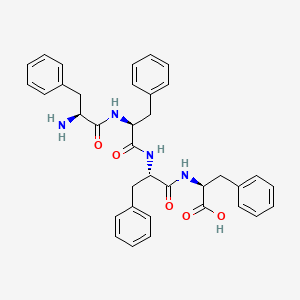
H-Phe-Phe-Phe-Phe-OH
Overview
Description
The compound H-Phe-Phe-Phe-Phe-OH is a tetrapeptide consisting of four phenylalanine residues. Phenylalanine is an essential amino acid that plays a crucial role in the biosynthesis of other amino acids and proteins. The tetrapeptide structure of this compound makes it an interesting subject for research in various fields, including chemistry, biology, and medicine.
Mechanism of Action
Target of Action
H-Phe-Phe-Phe-Phe-OH, also known as PHE-PHE-PHE-PHE ACETATE, is a peptide composed of four phenylalanine molecules . Phenylalanine is an essential amino acid and the precursor for the amino acid tyrosine
Mode of Action
The Phe-Phe motif, a key component of this compound, is known to drive the self-assembly of short peptides and their analogues into nanostructures and hydrogels . This self-assembly is a crucial aspect of the compound’s interaction with its targets.
Biochemical Pathways
The compound, being a peptide of phenylalanine, is involved in the biochemical pathways of phenylalanine and tyrosine . Phenylalanine is a precursor of catecholamines in the body . .
Result of Action
Molecules based on the phe-phe motif have found a range of applications in nanomedicine, from drug delivery and biomaterials to new therapeutic paradigms .
Biochemical Analysis
Biochemical Properties
H-Phe-Phe-Phe-Phe-OH: plays a significant role in biochemical reactions, particularly in the context of peptide self-assembly and molecular recognition. This tetrapeptide can interact with enzymes, proteins, and other biomolecules through non-covalent interactions such as hydrogen bonding, π-π stacking, and hydrophobic interactions. For instance, This compound has been shown to interact with DNA, potentially serving as a DNA molecular probe . Additionally, its aromatic nature allows it to participate in the formation of supramolecular structures, which can be utilized in various biochemical applications .
Cellular Effects
The effects of This compound on cellular processes are multifaceted. This compound can influence cell function by modulating cell signaling pathways, gene expression, and cellular metabolism. For example, This compound has been observed to affect the self-assembly of peptides, which can impact the structural integrity and function of cellular components . Moreover, its interaction with DNA suggests potential roles in gene regulation and expression .
Molecular Mechanism
At the molecular level, This compound exerts its effects through various binding interactions with biomolecules. The tetrapeptide can form stable complexes with DNA, proteins, and other macromolecules, influencing their activity and function. The self-assembly properties of This compound are driven by hydrogen bonding, van der Waals forces, and π-π interactions, which facilitate the formation of ordered structures . These interactions can lead to enzyme inhibition or activation, depending on the specific context and target biomolecule .
Temporal Effects in Laboratory Settings
In laboratory settings, the effects of This compound can change over time due to its stability and degradation properties. Studies have shown that this tetrapeptide can maintain its structural integrity under various conditions, but it may undergo degradation over extended periods . Long-term effects on cellular function have been observed in both in vitro and in vivo studies, indicating that This compound can have sustained impacts on cellular processes .
Dosage Effects in Animal Models
The effects of This compound in animal models vary with different dosages. At lower doses, the compound may exhibit beneficial effects on cellular function and metabolism. At higher doses, This compound can induce toxic or adverse effects, highlighting the importance of dosage optimization in experimental settings . Threshold effects have been observed, where specific concentrations of the tetrapeptide are required to elicit significant biological responses .
Metabolic Pathways
This compound: is involved in various metabolic pathways, interacting with enzymes and cofactors that facilitate its metabolism. The tetrapeptide can influence metabolic flux and alter metabolite levels, impacting overall cellular metabolism . Enzymes such as phenylalanine hydroxylase play a role in the metabolism of This compound , converting it into other bioactive compounds .
Transport and Distribution
Within cells and tissues, This compound is transported and distributed through interactions with specific transporters and binding proteins. These interactions can affect the localization and accumulation of the tetrapeptide, influencing its biological activity . The distribution of This compound within different cellular compartments can also impact its function and efficacy .
Subcellular Localization
The subcellular localization of This compound is determined by targeting signals and post-translational modifications that direct it to specific compartments or organelles. This localization can affect the activity and function of the tetrapeptide, as it interacts with various subcellular structures .
Preparation Methods
Synthetic Routes and Reaction Conditions
The synthesis of H-Phe-Phe-Phe-Phe-OH can be achieved through solid-phase peptide synthesis (SPPS). This method involves the sequential addition of protected amino acids to a growing peptide chain anchored to a solid resin. The process typically uses Fmoc (9-fluorenylmethyloxycarbonyl) protection for the amino groups and involves the following steps:
Coupling: The first amino acid is attached to the resin.
Deprotection: The Fmoc group is removed using a base such as piperidine.
Elongation: Subsequent amino acids are added one by one, with coupling agents like HBTU (O-Benzotriazole-N,N,N’,N’-tetramethyl-uronium-hexafluoro-phosphate) facilitating the formation of peptide bonds.
Cleavage: The completed peptide is cleaved from the resin using a strong acid like trifluoroacetic acid (TFA).
Industrial Production Methods
Industrial production of this compound can also involve biotechnological methods, such as recombinant DNA technology, where genetically modified organisms are used to produce the peptide in large quantities. Enzymatic catalysis is another method, where specific enzymes catalyze the formation of peptide bonds between phenylalanine residues.
Chemical Reactions Analysis
Types of Reactions
H-Phe-Phe-Phe-Phe-OH can undergo various chemical reactions, including:
Oxidation: The phenylalanine residues can be oxidized to form quinones.
Reduction: Reduction reactions can convert quinones back to phenols.
Substitution: The aromatic rings of phenylalanine can undergo electrophilic aromatic substitution reactions.
Common Reagents and Conditions
Oxidation: Reagents like potassium permanganate (KMnO₄) or hydrogen peroxide (H₂O₂) can be used.
Reduction: Reducing agents such as sodium borohydride (NaBH₄) or lithium aluminum hydride (LiAlH₄) are commonly employed.
Substitution: Electrophilic aromatic substitution can be facilitated by reagents like bromine (Br₂) or nitric acid (HNO₃).
Major Products Formed
Oxidation: Quinones and other oxidized derivatives.
Reduction: Phenols and reduced derivatives.
Substitution: Halogenated or nitrated phenylalanine derivatives.
Scientific Research Applications
H-Phe-Phe-Phe-Phe-OH has a wide range of applications in scientific research:
Chemistry: Used as a model compound for studying peptide synthesis and reactions.
Biology: Investigated for its role in protein-protein interactions and cellular signaling pathways.
Medicine: Explored for its potential therapeutic applications, including drug delivery systems and as a scaffold for developing new drugs.
Industry: Utilized in the development of biomaterials, such as hydrogels and nanostructures, for various industrial applications.
Comparison with Similar Compounds
Similar Compounds
H-Phe-Phe-OH: A dipeptide with two phenylalanine residues.
H-Phe-Phe-Phe-OH: A tripeptide with three phenylalanine residues.
H-Leu-Phe-Phe-OH: A tripeptide with leucine and two phenylalanine residues.
Uniqueness
H-Phe-Phe-Phe-Phe-OH is unique due to its tetrapeptide structure, which allows for more complex self-assembly and interactions compared to shorter peptides. Its ability to form stable nanostructures and hydrogels makes it particularly valuable for applications in nanomedicine and biomaterials.
Properties
IUPAC Name |
(2S)-2-[[(2S)-2-[[(2S)-2-[[(2S)-2-amino-3-phenylpropanoyl]amino]-3-phenylpropanoyl]amino]-3-phenylpropanoyl]amino]-3-phenylpropanoic acid | |
---|---|---|
Details | Computed by Lexichem TK 2.7.0 (PubChem release 2021.05.07) | |
Source | PubChem | |
URL | https://pubchem.ncbi.nlm.nih.gov | |
Description | Data deposited in or computed by PubChem | |
InChI |
InChI=1S/C36H38N4O5/c37-29(21-25-13-5-1-6-14-25)33(41)38-30(22-26-15-7-2-8-16-26)34(42)39-31(23-27-17-9-3-10-18-27)35(43)40-32(36(44)45)24-28-19-11-4-12-20-28/h1-20,29-32H,21-24,37H2,(H,38,41)(H,39,42)(H,40,43)(H,44,45)/t29-,30-,31-,32-/m0/s1 | |
Details | Computed by InChI 1.0.6 (PubChem release 2021.05.07) | |
Source | PubChem | |
URL | https://pubchem.ncbi.nlm.nih.gov | |
Description | Data deposited in or computed by PubChem | |
InChI Key |
NJNPEPZWJBIJCK-YDPTYEFTSA-N | |
Details | Computed by InChI 1.0.6 (PubChem release 2021.05.07) | |
Source | PubChem | |
URL | https://pubchem.ncbi.nlm.nih.gov | |
Description | Data deposited in or computed by PubChem | |
Canonical SMILES |
C1=CC=C(C=C1)CC(C(=O)NC(CC2=CC=CC=C2)C(=O)NC(CC3=CC=CC=C3)C(=O)NC(CC4=CC=CC=C4)C(=O)O)N | |
Details | Computed by OEChem 2.3.0 (PubChem release 2021.05.07) | |
Source | PubChem | |
URL | https://pubchem.ncbi.nlm.nih.gov | |
Description | Data deposited in or computed by PubChem | |
Isomeric SMILES |
C1=CC=C(C=C1)C[C@@H](C(=O)N[C@@H](CC2=CC=CC=C2)C(=O)N[C@@H](CC3=CC=CC=C3)C(=O)N[C@@H](CC4=CC=CC=C4)C(=O)O)N | |
Details | Computed by OEChem 2.3.0 (PubChem release 2021.05.07) | |
Source | PubChem | |
URL | https://pubchem.ncbi.nlm.nih.gov | |
Description | Data deposited in or computed by PubChem | |
Molecular Formula |
C36H38N4O5 | |
Details | Computed by PubChem 2.1 (PubChem release 2021.05.07) | |
Source | PubChem | |
URL | https://pubchem.ncbi.nlm.nih.gov | |
Description | Data deposited in or computed by PubChem | |
Molecular Weight |
606.7 g/mol | |
Details | Computed by PubChem 2.1 (PubChem release 2021.05.07) | |
Source | PubChem | |
URL | https://pubchem.ncbi.nlm.nih.gov | |
Description | Data deposited in or computed by PubChem | |
Retrosynthesis Analysis
AI-Powered Synthesis Planning: Our tool employs the Template_relevance Pistachio, Template_relevance Bkms_metabolic, Template_relevance Pistachio_ringbreaker, Template_relevance Reaxys, Template_relevance Reaxys_biocatalysis model, leveraging a vast database of chemical reactions to predict feasible synthetic routes.
One-Step Synthesis Focus: Specifically designed for one-step synthesis, it provides concise and direct routes for your target compounds, streamlining the synthesis process.
Accurate Predictions: Utilizing the extensive PISTACHIO, BKMS_METABOLIC, PISTACHIO_RINGBREAKER, REAXYS, REAXYS_BIOCATALYSIS database, our tool offers high-accuracy predictions, reflecting the latest in chemical research and data.
Strategy Settings
Precursor scoring | Relevance Heuristic |
---|---|
Min. plausibility | 0.01 |
Model | Template_relevance |
Template Set | Pistachio/Bkms_metabolic/Pistachio_ringbreaker/Reaxys/Reaxys_biocatalysis |
Top-N result to add to graph | 6 |
Feasible Synthetic Routes
Disclaimer and Information on In-Vitro Research Products
Please be aware that all articles and product information presented on BenchChem are intended solely for informational purposes. The products available for purchase on BenchChem are specifically designed for in-vitro studies, which are conducted outside of living organisms. In-vitro studies, derived from the Latin term "in glass," involve experiments performed in controlled laboratory settings using cells or tissues. It is important to note that these products are not categorized as medicines or drugs, and they have not received approval from the FDA for the prevention, treatment, or cure of any medical condition, ailment, or disease. We must emphasize that any form of bodily introduction of these products into humans or animals is strictly prohibited by law. It is essential to adhere to these guidelines to ensure compliance with legal and ethical standards in research and experimentation.