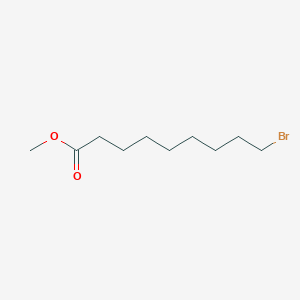
Methyl 9-bromononanoate
Overview
Description
Methyl 9-bromononanoate (C₁₀H₁₉BrO₂) is a brominated fatty acid ester characterized by a nine-carbon aliphatic chain with a bromine atom at the terminal (9th) position and a methyl ester group at the carboxyl end. This compound is primarily utilized in organic synthesis and pharmaceutical research, particularly in the development of lipid nanoparticles (LNPs) for drug delivery systems. Its synthesis involves the Hunsdiecker reaction, where the silver salt of methyl hydrogen sebacate is heated with bromine in carbon tetrachloride, yielding the brominated ester . The compound’s bromine atom enables nucleophilic substitution (SN2) reactions, making it a versatile intermediate for constructing complex molecules, such as ionizable lipids in LNPs .
Preparation Methods
Synthetic Routes and Reaction Conditions: Methyl 9-bromononanoate is typically synthesized through the esterification of 9-bromononanoic acid with methanol in the presence of a catalyst such as sulfuric acid . The reaction is carried out under reflux conditions to ensure complete conversion of the acid to the ester.
Industrial Production Methods: In an industrial setting, the production of this compound involves the same esterification process but on a larger scale. The reaction is conducted in a continuous flow reactor to optimize yield and efficiency. The product is then purified through distillation to remove any unreacted starting materials and by-products .
Chemical Reactions Analysis
Types of Reactions: Methyl 9-bromononanoate undergoes several types of chemical reactions, including:
Nucleophilic Substitution: The bromine atom can be replaced by various nucleophiles such as amines, thiols, and alkoxides.
Reduction: The ester group can be reduced to the corresponding alcohol using reducing agents like lithium aluminum hydride.
Hydrolysis: The ester can be hydrolyzed to 9-bromononanoic acid and methanol in the presence of a strong acid or base.
Common Reagents and Conditions:
Nucleophilic Substitution: Reagents like sodium azide or potassium thiocyanate in polar aprotic solvents.
Reduction: Lithium aluminum hydride in anhydrous ether.
Hydrolysis: Aqueous sodium hydroxide or hydrochloric acid under reflux.
Major Products Formed:
Nucleophilic Substitution: Various substituted nonanoates.
Reduction: 9-bromononan-1-ol.
Hydrolysis: 9-bromononanoic acid.
Scientific Research Applications
Methyl 9-bromononanoate has a wide range of applications in scientific research:
Chemistry: Used as a building block in the synthesis of more complex molecules.
Biology: Employed in the modification of biomolecules for studying biological processes.
Medicine: Investigated for its potential use in drug development and as a precursor for pharmaceuticals.
Industry: Utilized in the production of specialty chemicals and materials.
Mechanism of Action
The mechanism of action of methyl 9-bromononanoate involves its reactivity as an electrophile due to the presence of the bromine atom. This makes it susceptible to nucleophilic attack, leading to various substitution reactions. The ester group can also undergo hydrolysis or reduction, further expanding its reactivity profile .
Comparison with Similar Compounds
Comparison with Structurally Similar Compounds
Ethyl 9-Bromononanoate (CAS 28598-81-4)
Structural Differences: Ethyl 9-bromononanoate (C₁₁H₂₁BrO₂) replaces the methyl ester group with an ethyl group, increasing its molecular weight (265.19 g/mol vs. ~250.9 g/mol for the methyl analog) . Physical Properties:
- Boiling Point: 180°C at 30 mmHg (vs.
- Density : 1.17 g/cm³ .
- LogP: 3.68, indicating higher lipophilicity than the methyl ester due to the longer alkyl chain . Applications: Ethyl 9-bromononanoate is commercially available (purity >97%) and serves as a precursor in organic synthesis and pharmaceutical intermediates .
Methyl 8-Bromooctanoate (CAS 26825-92-3)
Structural Differences : This analog has an eight-carbon chain with bromine at the 8th position (C₉H₁₇BrO₂), reducing steric hindrance compared to the nine-carbon chain .
Physical Properties :
- Molecular Weight : 237.13 g/mol .
- Purity : >98% (commercially available) .
Reactivity : The shorter chain facilitates faster SN2 reactions due to reduced steric effects, making it preferable for synthesizing medium-chain derivatives .
Prenyl 9-Bromononanoate
Structural Differences: The prenyl (3-methyl-2-buten-1-ol) ester group introduces unsaturation, enhancing membrane fluidity in lipid-based formulations . Synthesis: Prepared via EDCI/DMAP-mediated esterification of 9-bromononanoic acid with prenol, yielding 76% efficiency . Applications: Used in stereoselective synthesis of nitro fatty acids, leveraging its unsaturated tail for improved solubility in hydrophobic environments .
Comparative Analysis Table
Key Research Findings
Synthetic Utility: this compound’s terminal bromine enables efficient SN2 reactions, as demonstrated in the synthesis of ionizable lipids for LNPs (75% yield in HBr-mediated acid conversion) .
Lipophilicity Trends: Ethyl 9-bromononanoate’s higher LogP (3.68 vs. ~3.2 for methyl) enhances its suitability for lipid-based formulations requiring prolonged tissue retention .
Steric Effects: Methyl 8-bromooctanoate’s shorter chain improves reaction kinetics in nucleophilic substitutions compared to its nine-carbon counterparts .
Functional Group Impact: Prenyl 9-bromononanoate’s unsaturated tail increases membrane fluidity, critical for endosomal escape in mRNA delivery systems .
Biological Activity
Methyl 9-bromononanoate (CAS Number: 67878-15-3) is an organic compound characterized by a bromine atom at the ninth carbon of a nonanoate chain. This unique structural configuration influences its biological activity, particularly in metabolic pathways and enzymatic interactions. This article provides a detailed overview of the biological activities associated with this compound, supported by data tables and relevant research findings.
- Molecular Formula : C10H19BrO2
- Molecular Weight : 251.16 g/mol
- Boiling Point : 131 °C at 2 Torr
- Purity : ≥ 97%
Biological Activity Overview
Preliminary studies indicate that this compound may influence various biochemical pathways, particularly those involving fatty acid metabolism. Notably, it has been shown to interact with Δ12-desaturase enzymes in microalgae, which are crucial for lipid metabolism. This interaction suggests potential applications in biotechnology and metabolic engineering .
Enzymatic Activity
The compound's impact on enzymatic activities is significant. This compound modulates the activity of desaturases, which are essential for the biosynthesis of unsaturated fatty acids. This modulation could have implications for understanding metabolic disorders and developing therapeutic agents targeting lipid metabolism .
Comparative Analysis with Similar Compounds
To understand the unique properties of this compound, it is beneficial to compare it with structurally similar compounds:
Compound Name | Molecular Formula | Unique Features |
---|---|---|
Methyl nonanoate | C9H18O2 | No halogen; simpler structure |
Methyl 8-bromooctanoate | C8H17BrO2 | Bromine at the eighth position |
Methyl 10-bromodecanoate | C11H23BrO2 | Bromine at the tenth position |
The placement of the bromine atom significantly alters the reactivity and biological activity of these compounds, making this compound particularly interesting for further research .
Study on Enzymatic Interaction
A study investigating the interaction between this compound and Δ12-desaturase enzymes demonstrated that this compound can enhance or inhibit the activity of these enzymes depending on concentration. The findings suggest that precise modulation of desaturase activity could lead to improved lipid profiles in organisms .
Q & A
Basic Research Questions
Q. What are the standard synthetic routes for preparing methyl 9-bromononanoate, and what critical reaction parameters must be controlled?
this compound is typically synthesized via esterification of 9-bromononanoic acid with methanol under acidic catalysis (e.g., H₂SO₄ or HCl). Key parameters include:
- Temperature : Maintain reflux conditions (≈70–80°C) to drive esterification without side reactions like hydrolysis .
- Solvent selection : Use anhydrous solvents (e.g., dichloromethane) to minimize water interference.
- Purification : Column chromatography or distillation is recommended to isolate the ester from unreacted acid or methanol . Note: Monitor reaction progress via thin-layer chromatography (TLC) and confirm purity using GC-MS or NMR .
Q. What safety protocols are essential when handling methyl 9-bromonanoate in laboratory settings?
- Hazard classification : Follow CLP Regulation (EC) No 1272/2008 guidelines for brominated compounds, which may include skin irritation, eye damage, or environmental toxicity .
- PPE : Use nitrile gloves, lab coats, and safety goggles. Conduct reactions in a fume hood to avoid inhalation .
- Waste disposal : Segregate halogenated waste and adhere to institutional protocols for brominated organics .
Q. How should researchers characterize this compound to confirm structural integrity?
- Spectroscopy :
- ¹H/¹³C NMR : Identify the methyl ester (δ ~3.6–3.7 ppm for OCH₃) and bromine-substituted carbon (δ ~30–40 ppm for C-Br) .
- GC-MS : Confirm molecular ion ([M⁺] at m/z 266) and fragmentation patterns (e.g., loss of CH₃OH or Br) .
Advanced Research Questions
Q. How can reaction conditions be optimized to improve the yield of this compound in large-scale syntheses?
- Catalyst screening : Compare Brønsted acids (e.g., p-toluenesulfonic acid) vs. Lewis acids (e.g., BF₃·OEt₂) for faster esterification .
- Solvent-free methods : Explore microwave-assisted synthesis to reduce reaction time and energy input .
- Statistical optimization : Use response surface methodology (RSM) to model variables like temperature, catalyst loading, and molar ratios .
Q. What analytical strategies resolve discrepancies in NMR data for this compound derivatives?
- Dynamic effects : Bromine’s quadrupolar moment may cause splitting artifacts. Use higher magnetic field strengths (≥400 MHz) or decoupling techniques .
- Impurity identification : Compare experimental ¹³C NMR shifts with computational predictions (e.g., DFT calculations) to detect regioisomers or unreacted precursors .
- Multi-technique validation : Cross-reference NMR with IR (C=O stretch at ~1740 cm⁻¹) and HPLC retention times .
Q. How does this compound perform in palladium-catalyzed cross-coupling reactions, and what mechanistic insights are critical?
- Substrate compatibility : Test coupling with arylboronic acids (Suzuki) or alkynes (Sonogashira). The bromine at C9 may sterically hinder transmetallation; use bulky ligands (e.g., XPhos) to enhance reactivity .
- Mechanistic studies : Monitor intermediates via in situ ³¹P NMR to assess ligand stability and oxidative addition rates .
- Byproduct analysis : Identify β-hydride elimination products (e.g., alkenes) via GC-MS to refine catalytic systems .
Q. Data Reporting and Methodological Rigor
Q. What statistical approaches ensure reproducibility in kinetic studies of this compound reactions?
- Error analysis : Report standard deviations (SD) for triplicate measurements. Use ANOVA to compare batch-to-batch variability .
- Dose-response modeling : Fit time-yield curves to first-order kinetics; justify significant digits based on instrument precision (e.g., ±0.1% for balances) .
Q. How should conflicting solubility data for this compound in polar solvents be addressed?
- Controlled experiments : Measure solubility in DMSO, methanol, and water at 25°C using gravimetric methods.
- Data reconciliation : Apply the Cohen’s d statistic to quantify effect sizes between studies. Discrepancies >10% warrant re-evaluation of solvent purity or temperature calibration .
Q. Tables for Key Data
Table 1. Comparative Analysis of Characterization Techniques
Technique | Parameters Measured | Precision/Tolerance | Evidence Source |
---|---|---|---|
¹H NMR | Chemical shifts, integration | ±0.01 ppm | |
GC-MS | Molecular ion, fragments | ±0.1 m/z | |
Elemental Analysis | %C, %H, %Br | ±0.3% |
Table 2. Optimization of Esterification Yield
Catalyst | Temp (°C) | Time (h) | Yield (%) |
---|---|---|---|
H₂SO₄ | 80 | 6 | 78 |
BF₃·OEt₂ | 70 | 4 | 85 |
p-TsOH | 75 | 5 | 82 |
Source: Adapted from |
Properties
IUPAC Name |
methyl 9-bromononanoate | |
---|---|---|
Details | Computed by LexiChem 2.6.6 (PubChem release 2019.06.18) | |
Source | PubChem | |
URL | https://pubchem.ncbi.nlm.nih.gov | |
Description | Data deposited in or computed by PubChem | |
InChI |
InChI=1S/C10H19BrO2/c1-13-10(12)8-6-4-2-3-5-7-9-11/h2-9H2,1H3 | |
Details | Computed by InChI 1.0.5 (PubChem release 2019.06.18) | |
Source | PubChem | |
URL | https://pubchem.ncbi.nlm.nih.gov | |
Description | Data deposited in or computed by PubChem | |
InChI Key |
UEWKXXYDRZHKCR-UHFFFAOYSA-N | |
Details | Computed by InChI 1.0.5 (PubChem release 2019.06.18) | |
Source | PubChem | |
URL | https://pubchem.ncbi.nlm.nih.gov | |
Description | Data deposited in or computed by PubChem | |
Canonical SMILES |
COC(=O)CCCCCCCCBr | |
Details | Computed by OEChem 2.1.5 (PubChem release 2019.06.18) | |
Source | PubChem | |
URL | https://pubchem.ncbi.nlm.nih.gov | |
Description | Data deposited in or computed by PubChem | |
Molecular Formula |
C10H19BrO2 | |
Details | Computed by PubChem 2.1 (PubChem release 2019.06.18) | |
Source | PubChem | |
URL | https://pubchem.ncbi.nlm.nih.gov | |
Description | Data deposited in or computed by PubChem | |
Molecular Weight |
251.16 g/mol | |
Details | Computed by PubChem 2.1 (PubChem release 2021.05.07) | |
Source | PubChem | |
URL | https://pubchem.ncbi.nlm.nih.gov | |
Description | Data deposited in or computed by PubChem | |
Synthesis routes and methods
Procedure details
Retrosynthesis Analysis
AI-Powered Synthesis Planning: Our tool employs the Template_relevance Pistachio, Template_relevance Bkms_metabolic, Template_relevance Pistachio_ringbreaker, Template_relevance Reaxys, Template_relevance Reaxys_biocatalysis model, leveraging a vast database of chemical reactions to predict feasible synthetic routes.
One-Step Synthesis Focus: Specifically designed for one-step synthesis, it provides concise and direct routes for your target compounds, streamlining the synthesis process.
Accurate Predictions: Utilizing the extensive PISTACHIO, BKMS_METABOLIC, PISTACHIO_RINGBREAKER, REAXYS, REAXYS_BIOCATALYSIS database, our tool offers high-accuracy predictions, reflecting the latest in chemical research and data.
Strategy Settings
Precursor scoring | Relevance Heuristic |
---|---|
Min. plausibility | 0.01 |
Model | Template_relevance |
Template Set | Pistachio/Bkms_metabolic/Pistachio_ringbreaker/Reaxys/Reaxys_biocatalysis |
Top-N result to add to graph | 6 |
Feasible Synthetic Routes
Disclaimer and Information on In-Vitro Research Products
Please be aware that all articles and product information presented on BenchChem are intended solely for informational purposes. The products available for purchase on BenchChem are specifically designed for in-vitro studies, which are conducted outside of living organisms. In-vitro studies, derived from the Latin term "in glass," involve experiments performed in controlled laboratory settings using cells or tissues. It is important to note that these products are not categorized as medicines or drugs, and they have not received approval from the FDA for the prevention, treatment, or cure of any medical condition, ailment, or disease. We must emphasize that any form of bodily introduction of these products into humans or animals is strictly prohibited by law. It is essential to adhere to these guidelines to ensure compliance with legal and ethical standards in research and experimentation.