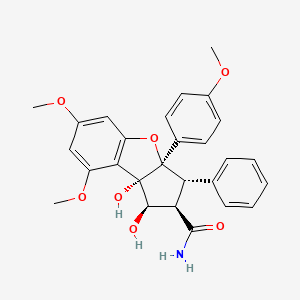
Didesmethylrocaglamide
Overview
Description
Didesmethylrocaglamide (DDR) is a cyclopenta[b]benzofuran lignan derived from plants of the Aglaia genus, structurally related to rocaglamide (Roc) but lacking two methyl groups . As a potent eukaryotic initiation factor 4A (eIF4A) inhibitor, DDR disrupts translation initiation by binding to eIF4A and preventing its interaction with mRNA, thereby suppressing the synthesis of oncogenic proteins critical for tumor survival and proliferation . DDR demonstrates nanomolar-range efficacy (IC50 values of 4–7 nM in canine osteosarcoma cells and 5–7 nM in human osteosarcoma cells) and induces apoptosis, G2/M cell cycle arrest, and DNA damage responses . Its stereochemistry is essential: the (-)-enantiomer is biologically active, whereas the (+)-enantiomer is 1,000-fold less potent .
Preparation Methods
Synthetic Routes and Reaction Conditions: The synthesis of didesmethylrocaglamide involves multiple steps, starting from the extraction of rocaglamide from Aglaia species. The synthetic route typically includes cyclization reactions, reduction processes, and functional group modifications to achieve the desired structure. Specific reaction conditions, such as temperature, solvents, and catalysts, are optimized to maximize yield and purity .
Industrial Production Methods: Industrial production of this compound is still in the experimental stage. The process involves large-scale extraction from plant sources, followed by chemical synthesis to refine and purify the compound. Advances in biotechnological methods, such as plant cell culture and genetic engineering, are being explored to enhance production efficiency .
Chemical Reactions Analysis
Types of Reactions: Didesmethylrocaglamide undergoes various chemical reactions, including:
Oxidation: This reaction involves the addition of oxygen or the removal of hydrogen, often using oxidizing agents like potassium permanganate or hydrogen peroxide.
Reduction: This reaction involves the addition of hydrogen or the removal of oxygen, typically using reducing agents like lithium aluminum hydride or sodium borohydride.
Substitution: This reaction involves the replacement of one functional group with another, often using reagents like halogens or nucleophiles.
Common Reagents and Conditions:
Oxidation: Potassium permanganate, hydrogen peroxide.
Reduction: Lithium aluminum hydride, sodium borohydride.
Substitution: Halogens, nucleophiles.
Major Products Formed: The major products formed from these reactions depend on the specific reagents and conditions used. For example, oxidation may yield hydroxylated derivatives, while reduction may produce deoxygenated compounds .
Scientific Research Applications
Cancer Treatment
Didesmethylrocaglamide has been extensively studied for its anti-cancer properties:
- Osteosarcoma : In both human and canine models, DDR has shown potent inhibition of osteosarcoma cell growth. Studies indicate that it is more effective than other rocaglates, with a significant reduction in tumor size observed in xenograft models .
- Other Tumors : The compound has also been evaluated against various sarcomas and leukemias, demonstrating broad-spectrum anti-tumor activity .
Combination Therapies
Research indicates that combining DDR with other therapeutic agents may enhance its efficacy. For instance:
- Synergistic Effects : When used alongside traditional chemotherapeutics, DDR may enhance overall treatment outcomes by targeting multiple pathways involved in tumor progression .
Preclinical Studies
Several studies have highlighted the efficacy of DDR in preclinical settings:
- A study published in Nature demonstrated that DDR significantly inhibited the growth of canine osteosarcoma patient-derived xenografts, suggesting its potential for translational applications in veterinary medicine .
- Another research article reported that DDR could effectively block tumor growth in various sarcoma cell lines, showcasing its versatility as an anti-cancer agent .
Clinical Relevance
While clinical trials are still necessary to fully establish the therapeutic window and safety profile of DDR, early findings are promising:
- The compound's mechanism as an eIF4A inhibitor aligns with ongoing clinical trials for related compounds like zotatifin (eFT226), which is currently being tested in adult malignancies .
Comparative Data Table
The following table summarizes the key findings related to this compound's applications across different studies:
Mechanism of Action
The anti-tumor activity of didesmethylrocaglamide is primarily driven by the inhibition of protein synthesis in tumor cells. This is achieved through the inhibition of prohibitin 1 and prohibitin 2, which are necessary for the proliferation of cancer cells. These proteins are implicated in the Ras-mediated CRaf-MEK-ERK signaling pathway responsible for phosphorylating eIF4E, a key factor in the initiation of protein synthesis. Additionally, rocaglamides can act directly on eIF4A, another translation initiation factor of the eIF4F complex .
Comparison with Similar Compounds
Structural and Functional Analogues
2.1.1 Rocaglamide (Roc)
- Structure-Activity Relationship (SAR): DDR shares Roc’s cyclopenta[b]benzofuran core but lacks methyl groups at C-1 and C-3. The hydroxyl group at C-8b is critical for activity; methylation at this position abolishes potency .
- Potency: DDR is 2–3 times more potent than Roc in inhibiting osteosarcoma cell growth (human IC50: 5–7 nM vs. 25–40 nM; canine IC50: 4–7 nM vs. 10–30 nM) .
- Mechanism: Both compounds reduce pro-survival kinases (e.g., AKT, ERK1/2) and induce p38-mediated stress responses, but DDR shows superior tumor growth inhibition in vivo (81% vs. 73% in canine PDX models) .
2.1.2 Silvestrol and Hybrid Derivatives
- Silvestrol : A flavagline with a dioxane ring, silvestrol inhibits eIF4A but requires higher doses (IC50 ~2–10 nM) and exhibits poor bioavailability .
- Hybrid DDR-Silvestrol (Compound 5) : A synthetic hybrid of DDR and silvestrol showed moderate activity (IC50 = 2.3 µM in HT-29 and PC-3 cells), suggesting that structural complexity may reduce efficacy compared to DDR .
2.1.3 Episilvestrol
- Episilvestrol, a silvestrol derivative, inhibits eIF4A but is less potent than DDR and Roc. Its mechanism overlaps with DDR but lacks evidence of efficacy in large-animal models .
Pharmacological and Preclinical Profiles
Table 1: Comparative IC50 Values in Osteosarcoma Models
Compound | Human Cell IC50 (nM) | Canine Cell IC50 (nM) | Key Structural Features |
---|---|---|---|
(-)-DDR | 5–7 | 4–7 | C-8b hydroxyl, demethylated C-1/C-5 |
(-)-Roc | 25–40 | 10–30 | C-8b hydroxyl, methylated C-1/C-5 |
(±)-DDR | 10–15 | 8–14 | Racemic mixture of DDR enantiomers |
(+)-DDR | 3,600–5,500 | N/A | Inactive enantiomer |
Table 2: In Vivo Efficacy in Canine PDX Models
Compound | Tumor Growth Inhibition (%) | Apoptosis Induction (TUNEL+ Cells) | Key Pathways Affected |
---|---|---|---|
(-)-DDR | 81 | 35–40% | p38, JUN, DNA damage response |
(-)-Roc | 73 | 25–30% | p38, AKT, ERK1/2 |
Silvestrol | ~60* | ~20%* | eIF4A, survivin |
*Data extrapolated from non-osteosarcoma studies .
Mechanistic Differentiation
- DDR vs. Roc : DDR’s demethylation enhances solubility and target engagement, leading to stronger suppression of IGF-1R and survivin . Both compounds activate p38, but DDR uniquely upregulates RHOB, a tumor suppressor linked to cytoskeletal remodeling .
- DDR vs. Silvestrol : Silvestrol’s dioxane ring improves RNA binding but increases off-target effects. DDR’s simpler structure may explain its broader therapeutic window .
Limitations and Challenges
- Resistance : NRF2 activation in cancer cells can counteract DDR’s effects by enhancing antioxidant responses .
Biological Activity
Didesmethylrocaglamide (DDR) is a compound belonging to the class of rocaglates, which have garnered attention for their potent biological activities, particularly in cancer therapy. This article provides a comprehensive overview of DDR's biological activity, focusing on its mechanisms of action, therapeutic potential, and relevant research findings.
DDR primarily exerts its biological effects through the inhibition of eukaryotic translation initiation factor 4A (eIF4A) , a critical component in the translation process. By inhibiting eIF4A, DDR disrupts the synthesis of various oncogenic proteins, leading to reduced tumor cell proliferation and induction of apoptosis.
Key Findings:
- Inhibition of Protein Synthesis : DDR has been shown to inhibit protein synthesis in tumor cells, which is central to its anti-tumor activity. This mechanism is similar to other rocaglamide derivatives .
- Induction of Apoptosis : Studies indicate that DDR treatment leads to increased cleavage of caspases and poly(ADP-ribose) polymerase (PARP), markers associated with apoptosis. This was observed in multiple cancer cell lines, including osteosarcoma and malignant peripheral nerve sheath tumors (MPNST) .
Anti-Tumor Activity
Research has demonstrated that DDR possesses significant anti-tumor effects across various cancer models. In particular, it has shown promise in treating osteosarcoma and other sarcomas.
Case Studies:
- Osteosarcoma Models : In both human and canine patient-derived xenograft models, DDR exhibited potent growth-inhibitory activity with IC50 values ranging from 20-35 nM, indicating strong efficacy against osteosarcoma cells .
- Ewing Sarcoma and Rhabdomyosarcoma : DDR also demonstrated effective suppression of growth in Ewing sarcoma and rhabdomyosarcoma cell lines, further supporting its broad anti-cancer potential .
Comparative Efficacy
A comparative analysis of DDR with other rocaglate compounds reveals its superior drug-like properties:
Compound | IC50 (nM) | Efficacy Against Osteosarcoma | Apoptosis Induction |
---|---|---|---|
This compound | 20-35 | High | Yes |
Rocaglamide | 10-12 | Moderate | Yes |
Silvestrol | >100 | Low | Limited |
Pharmacokinetics
Pharmacokinetic studies indicate that DDR has favorable absorption characteristics. Unlike silvestrol, which has limited oral bioavailability (<2%), DDR exhibits better pharmacokinetic profiles, making it a more viable candidate for oral administration .
Q & A
Q. Basic: What experimental methodologies are recommended for synthesizing Didesmethylrocaglamide and ensuring purity?
Answer:
Synthesis should follow validated protocols for Rocaglamide derivatives, emphasizing regioselective demethylation. Purification typically involves reversed-phase HPLC with UV detection (λ = 254 nm) and confirmation via high-resolution mass spectrometry (HRMS) and / NMR. Purity (>95%) must be verified using analytical HPLC with a C18 column (acetonitrile/water gradient) . For reproducibility, document solvent ratios, reaction temperatures, and catalyst concentrations. Detailed chromatograms and spectral data should be archived in supplementary materials .
Q. Basic: How can researchers confirm the structural identity of this compound post-synthesis?
Answer:
Combine spectroscopic techniques:
- NMR : Compare and chemical shifts with literature values for Rocaglamide derivatives, focusing on methyl group absence .
- HRMS : Confirm molecular ion [M+H] with <2 ppm mass accuracy.
- X-ray crystallography (if feasible): Resolve ambiguities in stereochemistry .
Report deviations from expected spectra and justify using computational simulations (e.g., DFT) if structural anomalies arise .
Q. Advanced: How should researchers address contradictory efficacy data for this compound across cell-based assays?
Answer:
Contradictions often stem from variability in:
- Cell lines : Use isogenic lines to control genetic background differences.
- Compound solubility : Pre-test in DMSO/vehicle and measure stability via LC-MS over 24h .
- Assay conditions : Standardize incubation times, serum concentrations, and control for off-target effects (e.g., ROS induction).
Perform meta-analyses of published data to identify confounding variables and apply multivariate regression models . Validate findings using orthogonal assays (e.g., CRISPR knockdown vs. pharmacological inhibition) .
Q. Advanced: What strategies optimize this compound’s bioavailability in pharmacokinetic studies?
Answer:
- Formulation : Use lipid-based nanoemulsions or cyclodextrin complexes to enhance aqueous solubility .
- Dosing regimens : Conduct pilot studies to determine optimal routes (IV vs. oral) and measure plasma half-life via LC-MS/MS.
- Metabolite profiling : Identify major Phase I/II metabolites using hepatocyte incubations and compare with in vivo samples .
Report bioavailability as absolute (F%) and relative to reference compounds, adjusting for protein binding using equilibrium dialysis .
Q. Basic: What in vitro assays are suitable for assessing this compound’s mechanism of action?
Answer:
- Enzyme inhibition : Use fluorescence-based assays (e.g., FLIPR) for real-time monitoring of target engagement (e.g., PRMT5/7 inhibition) .
- Cytotoxicity : Measure IC in cancer cell lines via MTT/WST-1 assays, normalizing to mitochondrial activity .
- Apoptosis markers : Quantify caspase-3/7 activation via luminescent substrates and confirm with Annexin V/PI flow cytometry .
Q. Advanced: How can computational modeling improve target prediction for this compound?
Answer:
- Molecular docking : Use AutoDock Vina or Schrödinger to screen against structural databases (e.g., PDB), prioritizing targets with ΔG < -8 kcal/mol .
- MD simulations : Validate binding stability over 100 ns trajectories (AMBER/CHARMM force fields).
- Network pharmacology : Integrate STRING or KEGG pathways to identify off-target effects and polypharmacological potential .
Cross-validate predictions with CRISPR-Cas9 knockout models .
Q. Basic: What statistical frameworks are critical for analyzing dose-response relationships?
Answer:
- Nonlinear regression : Fit data to sigmoidal models (e.g., Hill equation) using GraphPad Prism or R (drc package) .
- Error reporting : Include 95% confidence intervals for IC/EC values, not just p-values.
- Outlier detection : Apply Grubbs’ test or robust regression (e.g., Huber loss) to exclude non-physiological responses .
Q. Advanced: How can researchers resolve discrepancies in this compound’s reported cytotoxicity across studies?
Answer:
- Standardize protocols : Adopt CLSI guidelines for cell viability assays, including plate reader calibration and inter-lab replicates .
- Batch variability : Test multiple compound batches and report purity/certificates of analysis.
- Data normalization : Express cytotoxicity as fold-change relative to vehicle-treated controls, not absolute values .
Q. Basic: What analytical techniques validate this compound’s stability under experimental conditions?
Answer:
- Forced degradation : Expose to heat (40°C), light (ICH Q1B), and pH extremes (2–12), then quantify degradation products via UPLC-PDA .
- Long-term stability : Store at -80°C and test monthly for 6 months.
- Bioanalytical validation : Ensure LC-MS/MS methods meet FDA guidelines for accuracy (±15%) and precision (RSD <20%) .
Q. Advanced: How should researchers design studies to explore this compound’s synergies with existing therapies?
Answer:
- Combination indices : Calculate using Chou-Talalay or Bliss independence models .
- Mechanistic synergy : Use RNA-seq to identify co-targeted pathways (e.g., apoptosis/autophagy crosstalk).
- In vivo validation : Test combinations in PDX models with staggered dosing to mitigate toxicity .
Properties
IUPAC Name |
(1R,2R,3S,3aR,8bS)-1,8b-dihydroxy-6,8-dimethoxy-3a-(4-methoxyphenyl)-3-phenyl-2,3-dihydro-1H-cyclopenta[b][1]benzofuran-2-carboxamide | |
---|---|---|
Details | Computed by Lexichem TK 2.7.0 (PubChem release 2021.05.07) | |
Source | PubChem | |
URL | https://pubchem.ncbi.nlm.nih.gov | |
Description | Data deposited in or computed by PubChem | |
InChI |
InChI=1S/C27H27NO7/c1-32-17-11-9-16(10-12-17)27-22(15-7-5-4-6-8-15)21(25(28)30)24(29)26(27,31)23-19(34-3)13-18(33-2)14-20(23)35-27/h4-14,21-22,24,29,31H,1-3H3,(H2,28,30)/t21-,22-,24-,26+,27+/m1/s1 | |
Details | Computed by InChI 1.0.6 (PubChem release 2021.05.07) | |
Source | PubChem | |
URL | https://pubchem.ncbi.nlm.nih.gov | |
Description | Data deposited in or computed by PubChem | |
InChI Key |
RMNPQEWLGQURNX-PXIJUOARSA-N | |
Details | Computed by InChI 1.0.6 (PubChem release 2021.05.07) | |
Source | PubChem | |
URL | https://pubchem.ncbi.nlm.nih.gov | |
Description | Data deposited in or computed by PubChem | |
Canonical SMILES |
COC1=CC=C(C=C1)C23C(C(C(C2(C4=C(O3)C=C(C=C4OC)OC)O)O)C(=O)N)C5=CC=CC=C5 | |
Details | Computed by OEChem 2.3.0 (PubChem release 2021.05.07) | |
Source | PubChem | |
URL | https://pubchem.ncbi.nlm.nih.gov | |
Description | Data deposited in or computed by PubChem | |
Isomeric SMILES |
COC1=CC=C(C=C1)[C@]23[C@@H]([C@H]([C@H]([C@]2(C4=C(O3)C=C(C=C4OC)OC)O)O)C(=O)N)C5=CC=CC=C5 | |
Details | Computed by OEChem 2.3.0 (PubChem release 2021.05.07) | |
Source | PubChem | |
URL | https://pubchem.ncbi.nlm.nih.gov | |
Description | Data deposited in or computed by PubChem | |
Molecular Formula |
C27H27NO7 | |
Details | Computed by PubChem 2.1 (PubChem release 2021.05.07) | |
Source | PubChem | |
URL | https://pubchem.ncbi.nlm.nih.gov | |
Description | Data deposited in or computed by PubChem | |
Molecular Weight |
477.5 g/mol | |
Details | Computed by PubChem 2.1 (PubChem release 2021.05.07) | |
Source | PubChem | |
URL | https://pubchem.ncbi.nlm.nih.gov | |
Description | Data deposited in or computed by PubChem | |
Retrosynthesis Analysis
AI-Powered Synthesis Planning: Our tool employs the Template_relevance Pistachio, Template_relevance Bkms_metabolic, Template_relevance Pistachio_ringbreaker, Template_relevance Reaxys, Template_relevance Reaxys_biocatalysis model, leveraging a vast database of chemical reactions to predict feasible synthetic routes.
One-Step Synthesis Focus: Specifically designed for one-step synthesis, it provides concise and direct routes for your target compounds, streamlining the synthesis process.
Accurate Predictions: Utilizing the extensive PISTACHIO, BKMS_METABOLIC, PISTACHIO_RINGBREAKER, REAXYS, REAXYS_BIOCATALYSIS database, our tool offers high-accuracy predictions, reflecting the latest in chemical research and data.
Strategy Settings
Precursor scoring | Relevance Heuristic |
---|---|
Min. plausibility | 0.01 |
Model | Template_relevance |
Template Set | Pistachio/Bkms_metabolic/Pistachio_ringbreaker/Reaxys/Reaxys_biocatalysis |
Top-N result to add to graph | 6 |
Feasible Synthetic Routes
Disclaimer and Information on In-Vitro Research Products
Please be aware that all articles and product information presented on BenchChem are intended solely for informational purposes. The products available for purchase on BenchChem are specifically designed for in-vitro studies, which are conducted outside of living organisms. In-vitro studies, derived from the Latin term "in glass," involve experiments performed in controlled laboratory settings using cells or tissues. It is important to note that these products are not categorized as medicines or drugs, and they have not received approval from the FDA for the prevention, treatment, or cure of any medical condition, ailment, or disease. We must emphasize that any form of bodily introduction of these products into humans or animals is strictly prohibited by law. It is essential to adhere to these guidelines to ensure compliance with legal and ethical standards in research and experimentation.