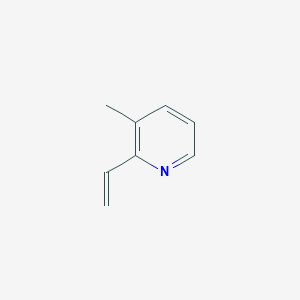
3-Methyl-2-vinylpyridine
Overview
Description
3-Methyl-2-vinylpyridine is an organic compound with the molecular formula C8H9N . It is a liquid at room temperature and is stored in an inert atmosphere, under -20°C . The compound has a molecular weight of 119.17 .
Synthesis Analysis
The synthesis of 2-methylpyridines, which are structurally similar to this compound, can be achieved through a simplified bench-top continuous flow setup . The reactions proceed with a high degree of selectivity, producing α-methylated pyridines .Molecular Structure Analysis
The molecular structure of 3-methyl 2-vinyl pyridinium phosphate (3M2VPP), a compound similar to this compound, has been optimized using Density Functional Theory . The theoretical structural parameters such as bond length, bond angle, and dihedral angle are determined by DFT methods .Chemical Reactions Analysis
Pyridinium salts, which include compounds like this compound, have been highlighted in terms of their synthetic routes and reactivity . They play an intriguing role in a wide range of research topics .Physical and Chemical Properties Analysis
This compound is a liquid at room temperature . The compound has a molecular weight of 119.17 . Theoretical vibrational, highest occupied molecular orbital - lowest unoccupied molecular orbital (HOMO-LUMO), natural bonding orbital (NBO), and electrostatic potential (ESP) analyses have also been performed .Scientific Research Applications
Catalytic Applications
3-Methyl-2-vinylpyridine has been utilized in various catalytic applications. For instance, poly(4-vinylpyridine) has been employed as a green, commercially available basic catalyst in the synthesis of benzopyrans and dihydropyranochromenes. This process offers advantages like mild reaction conditions, short reaction times, and high yields (Albadi, Mansournezhad, & Akbari Balout-Bangan, 2014). Additionally, polymer-protected palladium colloids involving poly-N-vinylpyrrolidone (PVP) have been used to catalyze H−D exchange between N-heterocyclic compounds and D2O, showing significant exchange at positions adjacent to the endocyclic nitrogen in various pyridines (Guy & Shapley, 2009).
Photovoltaic Applications
In photovoltaic research, a polymer blend formed by poly(3-hexylthiophene)-poly(4-vinylpyridine) block copolymers and phenyl-C61-butyric acid methyl ester has been reported, which demonstrates microphase separated morphologies of electron-donor and electron-acceptor rich domains, beneficial for photovoltaic devices (Sary et al., 2010).
Polymer Science
Studies in polymer science have explored the radical copolymerization of 3-vinylpyridine with other monomers, analyzing the reactivity ratios and providing insights into polymer chemistry (Trumbo, 1992). The electroconductivity and viscosity of complexes of poly(vinylpyridines) with alkali metal salts in organic solvents have also been investigated, revealing the ion-dipole binding mechanism of these polymers (Bekturganova, Dzhumadilov, & Bekturov, 1996).
Biomedical Applications
In biomedical research, quaternized poly(vinylpyridine) (PVP), known for its antimicrobial properties, has been studied for its hemocompatibility. Copolymerization with biocompatible polymers such as polyethyleneglycol methyl ether methacrylate and hydroxyethyl methacrylate was examined to improve biocompatibility (Allison, Applegate, & Youngblood, 2007).
Environmental Applications
This compound derivatives have been used in environmental applications, such as the efficient removal of methyl blue from aqueous solutions using poly(4-vinylpyridine)–graphene oxide–Fe3O4 magnetic nanocomposites. These composites exhibit high adsorption capacity, rapid adsorption rate, and good reusability (Li et al., 2019).
Safety and Hazards
Future Directions
Properties
IUPAC Name |
2-ethenyl-3-methylpyridine | |
---|---|---|
Details | Computed by LexiChem 2.6.6 (PubChem release 2019.06.18) | |
Source | PubChem | |
URL | https://pubchem.ncbi.nlm.nih.gov | |
Description | Data deposited in or computed by PubChem | |
InChI |
InChI=1S/C8H9N/c1-3-8-7(2)5-4-6-9-8/h3-6H,1H2,2H3 | |
Details | Computed by InChI 1.0.5 (PubChem release 2019.06.18) | |
Source | PubChem | |
URL | https://pubchem.ncbi.nlm.nih.gov | |
Description | Data deposited in or computed by PubChem | |
InChI Key |
VBXYZOUPVPNERZ-UHFFFAOYSA-N | |
Details | Computed by InChI 1.0.5 (PubChem release 2019.06.18) | |
Source | PubChem | |
URL | https://pubchem.ncbi.nlm.nih.gov | |
Description | Data deposited in or computed by PubChem | |
Canonical SMILES |
CC1=C(N=CC=C1)C=C | |
Details | Computed by OEChem 2.1.5 (PubChem release 2019.06.18) | |
Source | PubChem | |
URL | https://pubchem.ncbi.nlm.nih.gov | |
Description | Data deposited in or computed by PubChem | |
Molecular Formula |
C8H9N | |
Details | Computed by PubChem 2.1 (PubChem release 2019.06.18) | |
Source | PubChem | |
URL | https://pubchem.ncbi.nlm.nih.gov | |
Description | Data deposited in or computed by PubChem | |
Molecular Weight |
119.16 g/mol | |
Details | Computed by PubChem 2.1 (PubChem release 2021.05.07) | |
Source | PubChem | |
URL | https://pubchem.ncbi.nlm.nih.gov | |
Description | Data deposited in or computed by PubChem | |
Retrosynthesis Analysis
AI-Powered Synthesis Planning: Our tool employs the Template_relevance Pistachio, Template_relevance Bkms_metabolic, Template_relevance Pistachio_ringbreaker, Template_relevance Reaxys, Template_relevance Reaxys_biocatalysis model, leveraging a vast database of chemical reactions to predict feasible synthetic routes.
One-Step Synthesis Focus: Specifically designed for one-step synthesis, it provides concise and direct routes for your target compounds, streamlining the synthesis process.
Accurate Predictions: Utilizing the extensive PISTACHIO, BKMS_METABOLIC, PISTACHIO_RINGBREAKER, REAXYS, REAXYS_BIOCATALYSIS database, our tool offers high-accuracy predictions, reflecting the latest in chemical research and data.
Strategy Settings
Precursor scoring | Relevance Heuristic |
---|---|
Min. plausibility | 0.01 |
Model | Template_relevance |
Template Set | Pistachio/Bkms_metabolic/Pistachio_ringbreaker/Reaxys/Reaxys_biocatalysis |
Top-N result to add to graph | 6 |
Feasible Synthetic Routes
Disclaimer and Information on In-Vitro Research Products
Please be aware that all articles and product information presented on BenchChem are intended solely for informational purposes. The products available for purchase on BenchChem are specifically designed for in-vitro studies, which are conducted outside of living organisms. In-vitro studies, derived from the Latin term "in glass," involve experiments performed in controlled laboratory settings using cells or tissues. It is important to note that these products are not categorized as medicines or drugs, and they have not received approval from the FDA for the prevention, treatment, or cure of any medical condition, ailment, or disease. We must emphasize that any form of bodily introduction of these products into humans or animals is strictly prohibited by law. It is essential to adhere to these guidelines to ensure compliance with legal and ethical standards in research and experimentation.