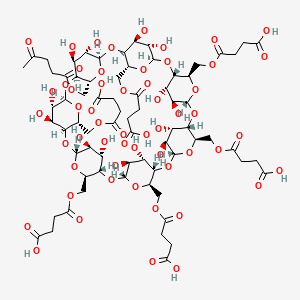
Succinyl-beta-cyclodextrin
Overview
Description
Succinyl-beta-cyclodextrin (Succinyl-β-CD) is a chemically modified derivative of beta-cyclodextrin (β-CD), characterized by the introduction of succinyl groups (-O-CO-CH₂-CH₂-COOH) at hydroxyl positions on the β-CD backbone. This substitution enhances its physicochemical properties, including aqueous solubility and host-guest complexation efficiency, making it a valuable excipient in pharmaceutical formulations . Succinyl-β-CD is particularly notable for its role in improving drug bioavailability by encapsulating hydrophobic molecules within its hydrophobic cavity while maintaining stability under physiological conditions . Its applications span drug delivery, chiral separations, and targeted therapeutics, with studies demonstrating its efficacy in enhancing the solubility of poorly water-soluble drugs such as curcumin and ethambutol .
Preparation Methods
Synthetic Routes and Reaction Conditions
Succinyl-beta-cyclodextrin is typically synthesized by reacting beta-cyclodextrin with succinic anhydride . The reaction is carried out in an aqueous medium, often with the addition of a base such as sodium hydroxide to facilitate the esterification process . The reaction conditions usually involve stirring the mixture at room temperature for several hours, followed by purification steps such as dialysis or precipitation to isolate the product .
Industrial Production Methods
Industrial production of this compound follows similar synthetic routes but on a larger scale. The process involves the use of industrial reactors and automated systems to control the reaction conditions precisely. The purification steps are also scaled up, often involving large-scale chromatography or filtration systems to ensure the purity of the final product .
Chemical Reactions Analysis
Types of Reactions
Succinyl-beta-cyclodextrin can undergo various chemical reactions, including:
Oxidation: This reaction can modify the hydroxyl groups on the cyclodextrin ring.
Reduction: This reaction can reduce any carbonyl groups present in the compound.
Substitution: This reaction can introduce different functional groups onto the cyclodextrin ring.
Common Reagents and Conditions
Oxidation: Common oxidizing agents include potassium permanganate and hydrogen peroxide.
Reduction: Common reducing agents include sodium borohydride and lithium aluminum hydride.
Substitution: Common reagents include alkyl halides and acyl chlorides.
Major Products Formed
The major products formed from these reactions depend on the specific reagents and conditions used. For example, oxidation can lead to the formation of carboxylated cyclodextrins, while substitution reactions can introduce various functional groups such as alkyl or acyl groups .
Scientific Research Applications
Pharmaceutical Applications
Drug Delivery Systems
SβCD has shown considerable promise as an excipient in drug formulation. Its capacity to enhance the solubility and bioavailability of poorly soluble drugs makes it a valuable component in oral dosage forms. For instance, research indicates that SβCD can form inclusion complexes with various drugs, improving their therapeutic efficacy and stability .
Case Study: Doxorubicin Delivery
A study demonstrated the use of SβCD in creating nanoparticles for the sustained release of doxorubicin, a chemotherapeutic agent. The nanoparticles exhibited improved biocompatibility and reduced side effects compared to free doxorubicin, indicating SβCD's potential in cancer therapy .
Chitosan/Succinyl-beta-Cyclodextrin Nanoparticles
Another innovative application involves the development of chitosan/SβCD nanoparticles designed for protein delivery. These nanoparticles provide a sustained release profile, enhancing the therapeutic effectiveness of protein-based drugs .
Environmental Applications
Uranium Extraction
SβCD has been utilized in the development of membranes for selective uranium (U(VI)) separation from aqueous solutions. A study reported that this compound-based membranes achieved a maximum adsorption capacity of 500 mg/g for U(VI) ions within 20 minutes, demonstrating high selectivity even in the presence of competing ions . This application is particularly relevant for environmental remediation efforts concerning radioactive contaminants.
Parameter | Value |
---|---|
Maximum Adsorption Capacity | 500 mg/g |
Equilibrium Time | 60 minutes |
Recovery Rate | 90% after 10 cycles |
Food Industry Applications
Food Preservation and Quality Enhancement
In food science, SβCD is used to improve the stability and sensory qualities of food products. It can encapsulate flavors and nutrients, protecting them from degradation due to oxidation or light exposure .
Case Study: Flavor Stabilization
Research has shown that SβCD can stabilize natural pigments such as curcumin and lycopene, enhancing their solubility and reducing oxidation rates. This property is beneficial for maintaining color and flavor in food products over extended storage periods .
Material Science Applications
Polymer Composites
SβCD can be incorporated into polymer matrices to create materials with enhanced properties. For example, studies have explored using SβCD in hydrogels and other polymeric systems to improve drug release profiles and mechanical strength .
Mechanism of Action
The mechanism of action of succinyl-beta-cyclodextrin involves its ability to form inclusion complexes with various guest molecules. The hydrophobic cavity of the cyclodextrin can encapsulate hydrophobic molecules, enhancing their solubility and stability in aqueous solutions . This property is particularly useful in drug delivery, where the inclusion complex can improve the bioavailability of poorly soluble drugs .
Comparison with Similar Compounds
Comparison with Similar Cyclodextrin Derivatives
Solubility and Substitution Effects
Succinyl-β-CD exhibits moderate to high water solubility, dependent on the degree of substitution (DS). Mono-, di-, and tri-succinyl derivatives show variable solubility profiles, with higher DS generally improving solubility due to increased ionization of carboxylate groups at physiological pH . In contrast:
- 2-Hydroxypropyl-beta-cyclodextrin (HP-β-CD) : Offers superior aqueous solubility (>500 mg/mL) due to hydroxypropyl groups, which prevent crystallization and enhance hydration .
- Methyl-beta-cyclodextrin (Methyl-β-CD) : Displays lower and inconsistent solubility owing to variable substitution patterns and batch-to-batch heterogeneity .
Table 1. Solubility and Substitution Characteristics
Derivative | Solubility (mg/mL) | Key Functional Group | DS Range |
---|---|---|---|
Succinyl-β-CD | 50–200* | Succinyl | 1–3 |
HP-β-CD | >500 | Hydroxypropyl | 4–8 |
Methyl-β-CD | 10–150 | Methyl | 10–15 |
S-β-CD | >1,000 | Sulfate | 7–14 |
*Data inferred from chiral separation studies .
Table 2. Toxicity Comparison
Derivative | Major Safety Concerns | Reversible Effects |
---|---|---|
Succinyl-β-CD | Low cytotoxicity (inferred) | N/A |
HP-β-CD | Diarrhea (oral), lung/kidney lesions (IV) | Yes |
Methyl-β-CD | Membrane disruption, batch variability | Partial |
S-β-CD | Anticoagulant activity | Dose-dependent |
Biological Activity
Succinyl-beta-cyclodextrin (Succinyl-β-CD) is a modified form of beta-cyclodextrin, a cyclic oligosaccharide known for its ability to form inclusion complexes with various guest molecules. The succinyl modification enhances its solubility and biological activity, making it a valuable compound in pharmaceutical and biomedical applications. This article explores the biological activity of Succinyl-β-CD, highlighting its mechanisms, applications, and relevant research findings.
Succinyl-β-CD exhibits several biological activities primarily due to its ability to form complexes with drugs and other bioactive compounds. This property enhances the solubility and stability of poorly soluble drugs, facilitating their delivery and absorption in biological systems.
- Drug Delivery : Succinyl-β-CD can encapsulate hydrophobic drugs, improving their bioavailability. This is particularly beneficial for anticancer agents, where enhanced solubility can lead to improved therapeutic efficacy.
- Antioxidant Activity : The modification with succinic acid may enhance the antioxidant properties of β-cyclodextrin, contributing to its protective effects against oxidative stress in cells.
- Anti-inflammatory Effects : Studies suggest that Succinyl-β-CD may exhibit anti-inflammatory properties, potentially beneficial in treating inflammatory diseases.
Case Studies and Research Findings
Several studies have investigated the biological activity of Succinyl-β-CD, demonstrating its potential in various applications:
1. Anticancer Activity
A study conducted on Succinyl-β-CD nanophotosensitizers showed significant anticancer effects against HeLa cervical cancer cells. The results indicated that these nanophotosensitizers had a lower IC50 value (1.05 mg/L) compared to the free chlorin e6 (Ce6), which had an IC50 greater than 5 mg/L. This suggests that Succinyl-β-CD enhances the efficacy of Ce6 in photodynamic therapy by improving cellular uptake and reducing toxicity to normal cells .
2. Food Industry Applications
Research has highlighted the use of cyclodextrins, including Succinyl-β-CD, in food applications to improve the solubility and stability of flavors and nutrients. The compound can prevent oxidation and enhance the sensory qualities of food products .
3. Toxicological Studies
Toxicological evaluations indicate that β-cyclodextrins have low acute oral toxicity and are generally recognized as safe for consumption within established acceptable daily intake levels (ADI) . Long-term studies have shown no evidence of carcinogenicity or genotoxicity associated with β-cyclodextrin derivatives.
Comparative Biological Activity Table
Biological Activity | Succinyl-β-CD | Standard β-CD |
---|---|---|
Solubility Enhancement | High | Moderate |
Antioxidant Activity | Moderate | Low |
Drug Delivery Efficiency | High | Moderate |
Toxicity | Low | Low |
Q & A
Q. Basic: What experimental methods are recommended for synthesizing and characterizing SBCD?
Methodological Answer:
Synthesis of SBCD involves succinylation of β-cyclodextrin using succinic anhydride under controlled alkaline conditions. Key parameters include reaction time, temperature, and molar ratios (e.g., 1:6 β-CD to succinic anhydride at 50°C for 12 hours). Post-synthesis, characterization should combine:
- Nuclear Magnetic Resonance (NMR): To confirm succinyl group attachment via shifts in -NMR (e.g., δ 2.5–2.7 ppm for succinyl protons) .
- High-Performance Liquid Chromatography (HPLC): To assess purity and degree of substitution (DS) using reverse-phase columns and UV detection .
- Fourier-Transform Infrared Spectroscopy (FTIR): To identify ester carbonyl stretches (~1730 cm) .
Table 1: Key Characterization Techniques
Technique | Parameter Analyzed | Example Data |
---|---|---|
-NMR | Substitution sites | δ 2.5–2.7 ppm (succinyl protons) |
HPLC | Purity/DS | Retention time = 8.2 min (DS 3.5) |
FTIR | Functional groups | 1730 cm (C=O stretch) |
Q. Basic: How can SBCD improve solubility of hydrophobic drugs, and what experimental designs validate this?
Methodological Answer:
SBCD enhances solubility via inclusion complex formation. To validate:
Phase Solubility Studies: Use the Higuchi-Connors method to determine stability constants () by plotting drug solubility vs. SBCD concentration .
Molecular Dynamics Simulations: Model guest-host interactions (e.g., van der Waals forces) to predict encapsulation efficiency .
In Vitro Dissolution Testing: Compare dissolution profiles of free drug vs. SBCD complexes in biorelevant media (e.g., FaSSGF) .
Q. Advanced: What mechanisms explain SBCD’s chiral recognition of drug stereoisomers?
Methodological Answer:
Chiral separation relies on stereoselective binding via:
- Cavity Size Matching: β-CD’s cavity accommodates aromatic rings, while succinyl groups enhance hydrophilicity and hydrogen bonding .
- Experimental Validation: Use chiral HPLC with SBCD-functionalized stationary phases. For example, resolve propranolol enantiomers by optimizing mobile phase pH (6.8–7.2) and acetonitrile content (15–20%) .
- 3D Structural Analysis: Compare X-ray crystallography or molecular docking results (e.g., warfarin’s failed resolution due to mismatched cavity interactions) .
Q. Advanced: How can researchers address contradictions in literature on SBCD’s solubility enhancement efficacy?
Methodological Answer:
Contradictions often arise from variability in DS, drug properties, or experimental conditions. Resolve via:
Meta-Analysis: Systematically compare studies using PRISMA guidelines. Stratify data by DS, drug logP, and temperature .
Sensitivity Testing: Replicate conflicting studies under standardized conditions (e.g., fixed pH, ionic strength) .
Multivariate Regression: Identify dominant factors (e.g., DS >3 improves solubility of high-logP drugs) .
Q. Advanced: What strategies optimize SBCD’s inclusion complex stability in multi-component drug formulations?
Methodological Answer:
Stability challenges in multi-component systems require:
Competitive Binding Assays: Use isothermal titration calorimetry (ITC) to measure binding affinities of SBCD with multiple drugs .
Co-Solvent Screening: Test excipients (e.g., polyethylene glycol) to reduce aggregation via steric stabilization .
Accelerated Stability Studies: Store complexes at 40°C/75% RH for 3 months and monitor drug release via HPLC .
Q. Basic: What analytical techniques confirm SBCD’s biocompatibility for in vivo applications?
Methodological Answer:
- Hemolysis Assay: Incubate SBCD with erythrocytes (1–10 mg/mL, 37°C/1 hour) and measure hemoglobin release at 540 nm .
- Cytotoxicity Testing: Use MTT assays on human cell lines (e.g., HepG2) with IC thresholds >1 mg/mL .
- In Vivo Toxicity: Administer SBCD (50–200 mg/kg) in rodent models and monitor liver/kidney function biomarkers (e.g., ALT, creatinine) .
Q. Advanced: How do thermodynamic vs. kinetic factors influence SBCD-drug complexation efficiency?
Methodological Answer:
- Thermodynamic Control: Measure via ITC; hydrophobic drugs (logP >3) favor spontaneous complexation () .
- Kinetic Trapping: Use rapid mixing (stopped-flow spectroscopy) to detect metastable complexes at high supersaturation .
- Temperature-Dependent Studies: Compare at 25°C vs. 37°C; entropy-driven binding dominates for rigid drugs .
Q. Basic: How to design a systematic review on SBCD’s applications in drug delivery?
Methodological Answer:
Define Scope: Use PICO framework (Population: hydrophobic drugs; Intervention: SBCD complexation; Comparison: other cyclodextrins; Outcomes: solubility, bioavailability) .
Search Strategy: Combine keywords (e.g., “succinyl-beta-cyclodextrin” AND “bioavailability”) in PubMed/Scopus, applying Boolean operators .
Data Extraction: Tabulate DS, drug class, and efficacy metrics (e.g., fold-increase in solubility) .
Q. Advanced: What computational tools predict SBCD’s binding affinity for novel drug candidates?
Methodological Answer:
- Molecular Docking (AutoDock Vina): Simulate drug orientation within SBCD’s cavity; prioritize ligands with binding energy <−6 kcal/mol .
- MD Simulations (GROMACS): Analyze complex stability over 100-ns trajectories (RMSD <2 Å indicates stable binding) .
- QSAR Modeling: Correlate drug descriptors (logP, polar surface area) with experimental values .
Q. Basic: What ethical guidelines apply when testing SBCD in preclinical models?
Methodological Answer:
- Institutional Approval: Submit protocols to IACUC for rodent studies, specifying dose ranges and humane endpoints .
- 3Rs Compliance: Replace in vivo tests with in vitro assays where possible; refine dosing to minimize animal use .
- Data Transparency: Report negative results (e.g., toxicity at high doses) to avoid publication bias .
Properties
IUPAC Name |
4-oxo-4-[[(1S,3R,5R,6S,8R,10R,11S,13R,15R,16S,18R,20R,21S,23R,25R,26S,28R,30R,31S,33R,35R,36S,37S,38S,39S,40S,41S,42S,43S,44S,45S,46S,47S,48S,49S)-10,15,20,25,30-pentakis(3-carboxypropanoyloxymethyl)-36,37,38,39,40,41,42,43,44,45,46,47,48,49-tetradecahydroxy-35-(4-oxopentanoyloxymethyl)-2,4,7,9,12,14,17,19,22,24,27,29,32,34-tetradecaoxaoctacyclo[31.2.2.23,6.28,11.213,16.218,21.223,26.228,31]nonatetracontan-5-yl]methoxy]butanoic acid | |
---|---|---|
Details | Computed by Lexichem TK 2.7.0 (PubChem release 2021.05.07) | |
Source | PubChem | |
URL | https://pubchem.ncbi.nlm.nih.gov | |
Description | Data deposited in or computed by PubChem | |
InChI |
InChI=1S/C71H100O55/c1-23(72)2-9-37(85)106-16-24-58-44(92)51(99)65(113-24)121-59-25(17-107-38(86)10-3-31(73)74)115-67(53(101)46(59)94)123-61-27(19-109-40(88)12-5-33(77)78)117-69(55(103)48(61)96)125-63-29(21-111-42(90)14-7-35(81)82)119-71(57(105)50(63)98)126-64-30(22-112-43(91)15-8-36(83)84)118-70(56(104)49(64)97)124-62-28(20-110-41(89)13-6-34(79)80)116-68(54(102)47(62)95)122-60-26(18-108-39(87)11-4-32(75)76)114-66(120-58)52(100)45(60)93/h24-30,44-71,92-105H,2-22H2,1H3,(H,73,74)(H,75,76)(H,77,78)(H,79,80)(H,81,82)(H,83,84)/t24-,25-,26-,27-,28-,29-,30-,44+,45+,46+,47+,48+,49+,50+,51+,52+,53+,54+,55+,56+,57+,58-,59-,60-,61-,62-,63-,64-,65-,66-,67-,68-,69-,70-,71-/m1/s1 | |
Details | Computed by InChI 1.0.6 (PubChem release 2021.05.07) | |
Source | PubChem | |
URL | https://pubchem.ncbi.nlm.nih.gov | |
Description | Data deposited in or computed by PubChem | |
InChI Key |
DIRLEDPEXJLCIL-JCWBWLHSSA-N | |
Details | Computed by InChI 1.0.6 (PubChem release 2021.05.07) | |
Source | PubChem | |
URL | https://pubchem.ncbi.nlm.nih.gov | |
Description | Data deposited in or computed by PubChem | |
Canonical SMILES |
CC(=O)CCC(=O)OCC1C2C(C(C(O1)OC3C(OC(C(C3O)O)OC4C(OC(C(C4O)O)OC5C(OC(C(C5O)O)OC6C(OC(C(C6O)O)OC7C(OC(C(C7O)O)OC8C(OC(O2)C(C8O)O)COC(=O)CCC(=O)O)COC(=O)CCC(=O)O)COC(=O)CCC(=O)O)COC(=O)CCC(=O)O)COC(=O)CCC(=O)O)COC(=O)CCC(=O)O)O)O | |
Details | Computed by OEChem 2.3.0 (PubChem release 2021.05.07) | |
Source | PubChem | |
URL | https://pubchem.ncbi.nlm.nih.gov | |
Description | Data deposited in or computed by PubChem | |
Isomeric SMILES |
CC(=O)CCC(=O)OC[C@@H]1[C@@H]2[C@H]([C@@H]([C@H](O1)O[C@@H]3[C@H](O[C@@H]([C@H]([C@@H]3O)O)O[C@@H]4[C@H](O[C@@H]([C@H]([C@@H]4O)O)O[C@@H]5[C@H](O[C@@H]([C@H]([C@@H]5O)O)O[C@@H]6[C@H](O[C@@H]([C@H]([C@@H]6O)O)O[C@@H]7[C@H](O[C@@H]([C@H]([C@@H]7O)O)O[C@@H]8[C@H](O[C@H](O2)[C@H]([C@@H]8O)O)COC(=O)CCC(=O)O)COC(=O)CCC(=O)O)COC(=O)CCC(=O)O)COC(=O)CCC(=O)O)COC(=O)CCC(=O)O)COC(=O)CCC(=O)O)O)O | |
Details | Computed by OEChem 2.3.0 (PubChem release 2021.05.07) | |
Source | PubChem | |
URL | https://pubchem.ncbi.nlm.nih.gov | |
Description | Data deposited in or computed by PubChem | |
Molecular Formula |
C71H100O55 | |
Details | Computed by PubChem 2.1 (PubChem release 2021.05.07) | |
Source | PubChem | |
URL | https://pubchem.ncbi.nlm.nih.gov | |
Description | Data deposited in or computed by PubChem | |
Molecular Weight |
1833.5 g/mol | |
Details | Computed by PubChem 2.1 (PubChem release 2021.05.07) | |
Source | PubChem | |
URL | https://pubchem.ncbi.nlm.nih.gov | |
Description | Data deposited in or computed by PubChem | |
Disclaimer and Information on In-Vitro Research Products
Please be aware that all articles and product information presented on BenchChem are intended solely for informational purposes. The products available for purchase on BenchChem are specifically designed for in-vitro studies, which are conducted outside of living organisms. In-vitro studies, derived from the Latin term "in glass," involve experiments performed in controlled laboratory settings using cells or tissues. It is important to note that these products are not categorized as medicines or drugs, and they have not received approval from the FDA for the prevention, treatment, or cure of any medical condition, ailment, or disease. We must emphasize that any form of bodily introduction of these products into humans or animals is strictly prohibited by law. It is essential to adhere to these guidelines to ensure compliance with legal and ethical standards in research and experimentation.