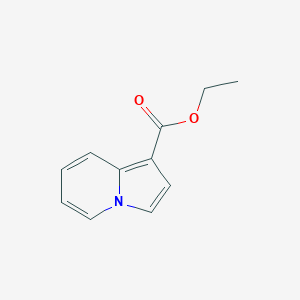
Ethyl Indolizine-1-carboxylate
Overview
Description
Ethyl Indolizine-1-carboxylate is an organic compound with the formula C11H11NO2 . It is used in the field of organic synthesis . It is also used in research and has potential biological activities .
Synthesis Analysis
Ethyl Indolizine-1-carboxylate can be synthesized through various methods. One method involves the use of sodium hydroxide in acetonitrile for 16 hours under heating conditions . Another method involves a multi-step reaction with SOCl2 in CH2Cl2 for 25 hours at 0 - 20 °C, followed by a reaction with NaOH in acetonitrile for 16 hours under heating .Molecular Structure Analysis
The molecular structure of Ethyl Indolizine-1-carboxylate consists of a pyrrole-type five-membered ring and a pyridine-type six-membered ring . The compound has a molecular weight of 189.21 .Chemical Reactions Analysis
Ethyl Indolizine-1-carboxylate can undergo various chemical reactions. For instance, it can participate in Pd-catalyzed reactions starting from pyrrole derivatives and 1,4-dibromo-1,3-butadienes .Physical And Chemical Properties Analysis
Ethyl Indolizine-1-carboxylate has several physical and chemical properties. It has a high GI absorption and is BBB permeant . It is also a CYP1A2 and CYP2C19 inhibitor . The compound has a Log Po/w (iLOGP) of 2.58 .Scientific Research Applications
- Researchers have explored ethyl indolizine-1-carboxylate derivatives for their antiviral potential. For instance:
- Indolizine derivatives have been screened for antitubercular effects:
- The indole scaffold (to which indolizine belongs) is widely used in drug development. For example:
Antiviral Activity
Antitubercular Activity
Drug Discovery
Safety and Hazards
Future Directions
Mechanism of Action
Target of Action
Ethyl Indolizine-1-carboxylate is a derivative of indole, a significant heterocyclic system found in natural products and drugs . Indole derivatives play a crucial role in cell biology and are used as biologically active compounds for the treatment of various disorders . They show various biologically vital properties and bind with high affinity to multiple receptors .
Mode of Action
Indole derivatives, in general, are known to interact with their targets, causing changes that can lead to various biological effects . The interaction of these compounds with their targets can lead to changes in cellular processes, potentially leading to therapeutic effects .
Biochemical Pathways
Indole derivatives can affect various biochemical pathways. For instance, they have been found to possess antiviral, anti-inflammatory, anticancer, anti-HIV, antioxidant, antimicrobial, antitubercular, antidiabetic, antimalarial, and anticholinesterase activities . These diverse biological activities suggest that Ethyl Indolizine-1-carboxylate may affect multiple biochemical pathways, leading to a broad range of downstream effects .
Result of Action
Given the broad spectrum of biological activities of indole derivatives, it is likely that ethyl indolizine-1-carboxylate could have multiple effects at the molecular and cellular levels .
properties
IUPAC Name |
ethyl indolizine-1-carboxylate | |
---|---|---|
Details | Computed by LexiChem 2.6.6 (PubChem release 2019.06.18) | |
Source | PubChem | |
URL | https://pubchem.ncbi.nlm.nih.gov | |
Description | Data deposited in or computed by PubChem | |
InChI |
InChI=1S/C11H11NO2/c1-2-14-11(13)9-6-8-12-7-4-3-5-10(9)12/h3-8H,2H2,1H3 | |
Details | Computed by InChI 1.0.5 (PubChem release 2019.06.18) | |
Source | PubChem | |
URL | https://pubchem.ncbi.nlm.nih.gov | |
Description | Data deposited in or computed by PubChem | |
InChI Key |
GDDGFXKIAMPUBV-UHFFFAOYSA-N | |
Details | Computed by InChI 1.0.5 (PubChem release 2019.06.18) | |
Source | PubChem | |
URL | https://pubchem.ncbi.nlm.nih.gov | |
Description | Data deposited in or computed by PubChem | |
Canonical SMILES |
CCOC(=O)C1=C2C=CC=CN2C=C1 | |
Details | Computed by OEChem 2.1.5 (PubChem release 2019.06.18) | |
Source | PubChem | |
URL | https://pubchem.ncbi.nlm.nih.gov | |
Description | Data deposited in or computed by PubChem | |
Molecular Formula |
C11H11NO2 | |
Details | Computed by PubChem 2.1 (PubChem release 2019.06.18) | |
Source | PubChem | |
URL | https://pubchem.ncbi.nlm.nih.gov | |
Description | Data deposited in or computed by PubChem | |
Molecular Weight |
189.21 g/mol | |
Details | Computed by PubChem 2.1 (PubChem release 2021.05.07) | |
Source | PubChem | |
URL | https://pubchem.ncbi.nlm.nih.gov | |
Description | Data deposited in or computed by PubChem | |
Product Name |
Ethyl Indolizine-1-carboxylate |
Retrosynthesis Analysis
AI-Powered Synthesis Planning: Our tool employs the Template_relevance Pistachio, Template_relevance Bkms_metabolic, Template_relevance Pistachio_ringbreaker, Template_relevance Reaxys, Template_relevance Reaxys_biocatalysis model, leveraging a vast database of chemical reactions to predict feasible synthetic routes.
One-Step Synthesis Focus: Specifically designed for one-step synthesis, it provides concise and direct routes for your target compounds, streamlining the synthesis process.
Accurate Predictions: Utilizing the extensive PISTACHIO, BKMS_METABOLIC, PISTACHIO_RINGBREAKER, REAXYS, REAXYS_BIOCATALYSIS database, our tool offers high-accuracy predictions, reflecting the latest in chemical research and data.
Strategy Settings
Precursor scoring | Relevance Heuristic |
---|---|
Min. plausibility | 0.01 |
Model | Template_relevance |
Template Set | Pistachio/Bkms_metabolic/Pistachio_ringbreaker/Reaxys/Reaxys_biocatalysis |
Top-N result to add to graph | 6 |
Feasible Synthetic Routes
Disclaimer and Information on In-Vitro Research Products
Please be aware that all articles and product information presented on BenchChem are intended solely for informational purposes. The products available for purchase on BenchChem are specifically designed for in-vitro studies, which are conducted outside of living organisms. In-vitro studies, derived from the Latin term "in glass," involve experiments performed in controlled laboratory settings using cells or tissues. It is important to note that these products are not categorized as medicines or drugs, and they have not received approval from the FDA for the prevention, treatment, or cure of any medical condition, ailment, or disease. We must emphasize that any form of bodily introduction of these products into humans or animals is strictly prohibited by law. It is essential to adhere to these guidelines to ensure compliance with legal and ethical standards in research and experimentation.