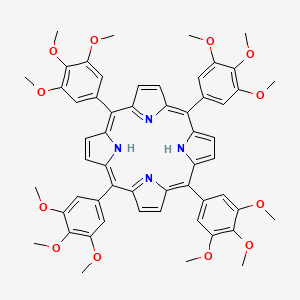
5,10,15,20-Tetra(3,4,5-trimethoxyphenyl)-21H,23H-porphine
Overview
Description
5,10,15,20-Tetra(3,4,5-trimethoxyphenyl)-21H,23H-porphine, also known as TTOP, is a type of porphyrin that has been studied for its self-assembly and photocatalytic activity . It is well dispersed as monomers in tetrahydrofuran (THF), but it stacks into aggregates upon the addition of water . The compound is available for experimental and research use .
Synthesis Analysis
The synthesis of TTOP has been studied in the context of its self-assembly and photocatalytic activity . It was found that TTOP can be dispersed as monomers in THF, but it forms aggregates when water is added . The formation and control of these aggregates are influenced by the solvent, which plays a crucial role in the effectiveness of TTOP as a porphyrin-based photocatalyst .Molecular Structure Analysis
The molecular structure of TTOP has been confirmed through various spectroscopic methods . The compound has been observed to form different nanostructures depending on the mixture of THF and water . For instance, microrods and sphere-like particles were obtained in 70% water in THF, while particles with irregular shapes were obtained at 90% water in THF .Chemical Reactions Analysis
The chemical reactions involving TTOP have been investigated in terms of its photocatalytic activity . The compound has been found to exhibit significant photocatalytic ability for the degradation of dyes . The rate constants of dye degradation were found to vary depending on the aggregate states of TTOP .Physical And Chemical Properties Analysis
TTOP has been characterized by its physical and chemical properties, including its ability to form different nanostructures and its photocatalytic activity . The compound’s properties are influenced by the solvent, which affects its self-assembly into aggregates .Scientific Research Applications
Photocatalytic Behaviour and Nanostructure Control
The compound has been studied for its photocatalytic behaviour and the ability to control its nanostructure . In a study, the self-assembly of this compound in a mixture of tetrahydrofuran (THF) and H2O was investigated, and the photocatalytic activity of the resulting self-assembled aggregates toward the degradation of organic compounds was examined . The study found that depending on the THF/H2O mixture, various nanostructures were observed .
Degradation of Organic Compounds
The compound has been used in the degradation of organic compounds . The study found that the compound was well dispersed as monomers in THF, however, it stacked into aggregates upon the addition of water (20–90%) . These structures exhibited a significant difference in photocatalytic ability for the degradation of dye .
Antimicrobial Photodynamic Therapy
The compound has potential applications in antimicrobial photodynamic therapy . Photodynamic therapy is a treatment that uses a drug, called a photosensitizer or photosensitizing agent, and a particular type of light to kill cancer cells .
Treatment of Ischemic Heart Disease and Cerebral Palsy
Cationic porphyrins, including 5,10,15,20-Tetra(3,4,5-trimethoxyphenyl)porphyrin, have been predicted to have potential use as drugs for the treatment of ischemic heart disease and cerebral palsy .
Mechanism of Action
Target of Action
The primary target of 5,10,15,20-Tetra(3,4,5-trimethoxyphenyl)porphyrin (TTOP) is organic compounds . TTOP is used in the degradation of these compounds through a photocatalytic process .
Mode of Action
TTOP interacts with its targets through a process known as solvophobic supramolecular self-assembly . In this process, TTOP is dispersed as monomers in tetrahydrofuran (THF), and it forms aggregates upon the addition of water . These aggregates then interact with the organic compounds, leading to their degradation .
Biochemical Pathways
The degradation of organic compounds by TTOP involves photocatalytic pathways . The photocatalytic activity of TTOP is influenced by the formation and control of porphyrin aggregates, which are crucial to an effective porphyrin-based photocatalyst .
Pharmacokinetics
The formation of ttop aggregates in different solvent conditions suggests that its bioavailability may be influenced by the solvent environment .
Result of Action
The result of TTOP’s action is the degradation of organic compounds . The rate of degradation varies depending on the state of TTOP (monomer or aggregate) and the percentage of water in THF .
Action Environment
The action of TTOP is significantly influenced by the solvent environment . The formation of different nanostructures and the photocatalytic ability of TTOP are affected by the mixture of THF and water . For instance, microrods and sphere-like particles are formed in 70% water in THF, while particles with irregular shapes connecting into large aggregates are obtained at 90% water in THF .
Future Directions
The future directions for the study of TTOP could involve further investigation of its self-assembly and photocatalytic activity . The role of the solvent in the formation and control of TTOP aggregates could be a particular area of focus . Additionally, the compound’s potential applications in areas such as solar energy and photodynamic cancer therapy could be explored .
properties
IUPAC Name |
5,10,15,20-tetrakis(3,4,5-trimethoxyphenyl)-21,23-dihydroporphyrin | |
---|---|---|
Details | Computed by Lexichem TK 2.7.0 (PubChem release 2021.05.07) | |
Source | PubChem | |
URL | https://pubchem.ncbi.nlm.nih.gov | |
Description | Data deposited in or computed by PubChem | |
InChI |
InChI=1S/C56H54N4O12/c1-61-41-21-29(22-42(62-2)53(41)69-9)49-33-13-15-35(57-33)50(30-23-43(63-3)54(70-10)44(24-30)64-4)37-17-19-39(59-37)52(32-27-47(67-7)56(72-12)48(28-32)68-8)40-20-18-38(60-40)51(36-16-14-34(49)58-36)31-25-45(65-5)55(71-11)46(26-31)66-6/h13-28,57,60H,1-12H3 | |
Details | Computed by InChI 1.0.6 (PubChem release 2021.05.07) | |
Source | PubChem | |
URL | https://pubchem.ncbi.nlm.nih.gov | |
Description | Data deposited in or computed by PubChem | |
InChI Key |
WVYQOJACJYPRCF-UHFFFAOYSA-N | |
Details | Computed by InChI 1.0.6 (PubChem release 2021.05.07) | |
Source | PubChem | |
URL | https://pubchem.ncbi.nlm.nih.gov | |
Description | Data deposited in or computed by PubChem | |
Canonical SMILES |
COC1=CC(=CC(=C1OC)OC)C2=C3C=CC(=C(C4=NC(=C(C5=CC=C(N5)C(=C6C=CC2=N6)C7=CC(=C(C(=C7)OC)OC)OC)C8=CC(=C(C(=C8)OC)OC)OC)C=C4)C9=CC(=C(C(=C9)OC)OC)OC)N3 | |
Details | Computed by OEChem 2.3.0 (PubChem release 2021.05.07) | |
Source | PubChem | |
URL | https://pubchem.ncbi.nlm.nih.gov | |
Description | Data deposited in or computed by PubChem | |
Molecular Formula |
C56H54N4O12 | |
Details | Computed by PubChem 2.1 (PubChem release 2021.05.07) | |
Source | PubChem | |
URL | https://pubchem.ncbi.nlm.nih.gov | |
Description | Data deposited in or computed by PubChem | |
DSSTOX Substance ID |
DTXSID001248841 | |
Record name | meso-Tetrakis(3,4,5-trimethoxyphenyl)porphyrin | |
Source | EPA DSSTox | |
URL | https://comptox.epa.gov/dashboard/DTXSID001248841 | |
Description | DSSTox provides a high quality public chemistry resource for supporting improved predictive toxicology. | |
Molecular Weight |
975.0 g/mol | |
Details | Computed by PubChem 2.1 (PubChem release 2021.05.07) | |
Source | PubChem | |
URL | https://pubchem.ncbi.nlm.nih.gov | |
Description | Data deposited in or computed by PubChem | |
CAS RN |
74684-35-8 | |
Record name | meso-Tetrakis(3,4,5-trimethoxyphenyl)porphyrin | |
Source | EPA DSSTox | |
URL | https://comptox.epa.gov/dashboard/DTXSID001248841 | |
Description | DSSTox provides a high quality public chemistry resource for supporting improved predictive toxicology. | |
Retrosynthesis Analysis
AI-Powered Synthesis Planning: Our tool employs the Template_relevance Pistachio, Template_relevance Bkms_metabolic, Template_relevance Pistachio_ringbreaker, Template_relevance Reaxys, Template_relevance Reaxys_biocatalysis model, leveraging a vast database of chemical reactions to predict feasible synthetic routes.
One-Step Synthesis Focus: Specifically designed for one-step synthesis, it provides concise and direct routes for your target compounds, streamlining the synthesis process.
Accurate Predictions: Utilizing the extensive PISTACHIO, BKMS_METABOLIC, PISTACHIO_RINGBREAKER, REAXYS, REAXYS_BIOCATALYSIS database, our tool offers high-accuracy predictions, reflecting the latest in chemical research and data.
Strategy Settings
Precursor scoring | Relevance Heuristic |
---|---|
Min. plausibility | 0.01 |
Model | Template_relevance |
Template Set | Pistachio/Bkms_metabolic/Pistachio_ringbreaker/Reaxys/Reaxys_biocatalysis |
Top-N result to add to graph | 6 |
Feasible Synthetic Routes
Q & A
Q1: What is the impact of solvent on the self-assembly and photocatalytic activity of 5,10,15,20-Tetra(3,4,5-trimethoxyphenyl)porphyrin (TTOP)?
A1: The solvent plays a crucial role in controlling the self-assembly of TTOP and consequently impacting its photocatalytic activity. Research has shown that TTOP remains in a monomeric state in tetrahydrofuran (THF) but forms various nanostructures when water is gradually introduced into the solution [, ]. For instance, in a 70:30 THF/water mixture, TTOP assembles into microrods and sphere-like particles. Increasing the water content to 80% transforms these structures into slabs and irregular aggregates at 90% water. This change in morphology significantly affects the photocatalytic degradation rate of dyes like Rhodamine B (RhB) and Methylene Blue (MB). The degradation rate constant of RhB increased with increasing water content, highlighting the influence of self-assembled structures on photocatalytic efficiency [].
Q2: How does bromination affect the properties of tetraarylporphyrins like TTOP?
A2: Bromination of tetraarylporphyrins can occur selectively at different positions depending on the substituents present and reaction conditions []. In the case of TTOP or similar compounds, bromination can lead to octabromination at the 2,6-positions of the meso-aryl substituents. This structural modification significantly impacts the electrochemical properties of the porphyrin. For example, in the cited research, 5,10,15,20-tetrakis(2,6-dibromo-3,4,5-trimethoxyphenyl)porphyrin exhibited distinct electrochemical behavior compared to its non-brominated counterpart [].
Q3: Can you provide details on the structural characteristics of TTOP?
A3: 5,10,15,20-Tetra(3,4,5-trimethoxyphenyl)porphyrin (TTOP), also referred to as 5,10,15,20-tetrakis(3,4,5-trimethoxyphenyl)porphyrin or 5,10,15,20-Tetra(3,4,5-trimethoxyphenyl)-21H,23H-porphine, is a symmetrically substituted porphyrin derivative. While the provided research excerpts don't explicitly state the molecular formula and weight, they can be deduced from the structure. TTOP features four 3,4,5-trimethoxyphenyl groups attached to the meso positions of the porphyrin ring. Characterization data, including spectroscopic details like UV-Vis absorption and fluorescence emission, are essential for understanding its photophysical properties.
Q4: Are there any studies on the environmental impact or degradation of TTOP and its derivatives?
A4: The provided research excerpts do not offer specific information about the environmental impact, degradation pathways, or ecotoxicological effects of TTOP or its brominated derivatives. Further investigation is necessary to evaluate the potential risks and develop strategies for the responsible handling and disposal of these compounds.
Q5: What are the potential applications of TTOP in material science?
A5: The self-assembly behavior of TTOP in different solvent mixtures makes it a promising candidate for developing nanostructured materials [, ]. The morphology control achieved by adjusting solvent ratios allows for the creation of distinct nanostructures, each potentially possessing unique properties. This opens avenues for applications in areas like sensing, optoelectronics, and catalysis, where controlled self-assembly and tunable properties are highly desirable.
Disclaimer and Information on In-Vitro Research Products
Please be aware that all articles and product information presented on BenchChem are intended solely for informational purposes. The products available for purchase on BenchChem are specifically designed for in-vitro studies, which are conducted outside of living organisms. In-vitro studies, derived from the Latin term "in glass," involve experiments performed in controlled laboratory settings using cells or tissues. It is important to note that these products are not categorized as medicines or drugs, and they have not received approval from the FDA for the prevention, treatment, or cure of any medical condition, ailment, or disease. We must emphasize that any form of bodily introduction of these products into humans or animals is strictly prohibited by law. It is essential to adhere to these guidelines to ensure compliance with legal and ethical standards in research and experimentation.