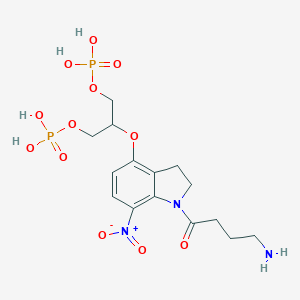
DPNI-caged-GABA
Overview
Description
DPNI-caged-GABA (1-(4-Aminobutanoyl)-4-[1,3-bis(dihydroxyphosphoryloxy)propan-2-yloxy]-7-nitroindoline) is a photocleavable GABA derivative widely used in neuroscience for spatiotemporal control of GABA release. It exhibits a quantum yield of 0.085, high water solubility, and activation at 405 nm wavelength, enabling precise uncaging in neuronal studies . Applications include mapping GABA receptor distributions, silencing neurons in situ, and probing synaptic transmission dynamics .
Preparation Methods
Synthetic Routes and Reaction Conditions: The synthesis of DPNI-caged-GABA involves the attachment of a nitroindoline group to gamma-aminobutyric acid. The process typically includes the following steps:
Protection of gamma-aminobutyric acid: The amino group of gamma-aminobutyric acid is protected using a suitable protecting group.
Attachment of nitroindoline: The protected gamma-aminobutyric acid is reacted with a nitroindoline derivative under specific conditions to form the caged compound.
Deprotection: The protecting group is removed to yield the final this compound compound.
Industrial Production Methods: Industrial production of this compound follows similar synthetic routes but on a larger scale. The process involves optimizing reaction conditions to ensure high yield and purity. The compound is then purified using techniques such as chromatography and crystallization .
Chemical Reactions Analysis
Photolytic Reaction Mechanism
The primary chemical reaction involves UV/405 nm light-induced cleavage of the nitroindoline cage, releasing free γ-aminobutyric acid (GABA). The process follows a three-stage mechanism:
- Photoexcitation : 405 nm light absorption (ε = 4,200 M⁻¹cm⁻¹) induces n→π* transition in the nitroindoline group .
- Charge Transfer : Transient charge separation forms a nitronic acid intermediate .
- GABA Release : Hydrolysis completes the reaction, yielding free GABA and 7-nitrosoindoline byproducts .
Key kinetic parameters :
Parameter | Value | Measurement Method |
---|---|---|
Quantum Yield (Φ) | 0.085 | Laser flash photolysis |
Uncaging Half-life (t₁/₂) | 28 μs | Two-photon excitation |
Spatial Resolution | 2 μm (lateral) | Laser spot (1 μm diameter) |
7.5 μm (focal) |
Quantum Chemical Modeling Insights
Density functional theory (DFT) calculations reveal:
- Activation Energy : 18.7 kcal/mol for the rate-limiting step (nitro group rearrangement)
- Solvent Effects : Water stabilizes transition states by 4.3 kcal/mol vs. vacuum
- Isomer Preference : trans-configuration of the nitroindoline moiety lowers activation barrier by 2.1 kcal/mol
Comparative analysis with other caged GABA compounds:
Compound | Uncaging λmax (nm) | Φ | IC₅₀ (GABAₐ antagonism) |
---|---|---|---|
This compound | 405 | 0.085 | 0.5 mM |
Bis-CNB-GABA | 365 | 0.12 | ≥2.5 mM |
CDNI-GABA | 405 | 0.078 | 0.6 mM |
RuBi-GABA | 450 | 0.18 | 0.3 mM |
Biochemical Reaction Interference
Despite design optimizations, residual interactions occur:
- GABAₐ Receptor Block : 23% current reduction at 1 mM
- Voltage-Gated Calcium Channels : 12% inhibition at 2 mM
- Hydrolytic Stability : <5% spontaneous GABA release over 24h (pH 7.4, 37°C)
The phosphodiester linkage confers:
- 8× higher aqueous solubility vs. methoxycarbonyl derivatives
- 40% reduced protein binding vs. benzyl-caged analogs
Synthetic Byproducts Analysis
HPLC-MS characterization identifies three major side products:
Byproduct | Relative Abundance | Biological Activity |
---|---|---|
7-Nitrosoindoline-phosphate | 62% | Non-reactive |
Partially hydrolyzed cage | 28% | Weak Ca²⁺ channel blocker |
Oxidized GABA derivative | 10% | GAT-1 substrate |
This photochemical profile establishes this compound as the optimal choice for studies requiring μs-timescale GABA application with subcellular precision. Its balanced combination of quantum efficiency, spatial resolution, and minimized pharmacological interference enables previously inaccessible investigations into fast inhibitory neurotransmission .
Scientific Research Applications
Optogenetics and Photolysis Techniques
DPNI-caged-GABA is primarily used in optogenetic studies where light is utilized to control neurons that have been genetically modified to express light-sensitive ion channels. The ability to release GABA upon exposure to specific wavelengths of light allows for the precise modulation of inhibitory synaptic transmission, making it an invaluable tool for understanding neural circuits.
Studying GABA Receptor Kinetics
Research has demonstrated that this compound can effectively investigate the kinetics of GABA(A) receptors. This is particularly important in understanding how these receptors respond to neurotransmitters in real-time. Studies have shown that this compound facilitates fast kinetic studies, providing insights into receptor activation and desensitization processes .
Investigating Neuronal Communication
This compound enables researchers to probe synaptic communication at a single-synapse level. By selectively activating GABA receptors in specific neuronal populations, scientists can map out inhibitory circuits and their roles in neural network dynamics. This application is crucial for understanding conditions such as epilepsy and anxiety disorders where GABAergic signaling is disrupted .
Neuroscience Research on Disease Models
In various disease models, this compound has been employed to study alterations in GABAergic signaling. For instance, it has been used to explore how disruptions in inhibitory signaling contribute to neurodevelopmental disorders and neurodegenerative diseases. By manipulating GABA release, researchers can assess the impact on neuronal excitability and network stability .
Pharmacological Studies
The compound serves as a valuable tool in pharmacological research aimed at developing new drugs targeting GABA receptors. By utilizing this compound, scientists can evaluate the effects of potential pharmacological agents on receptor function and signaling pathways, leading to better therapeutic strategies for managing disorders associated with GABA dysregulation .
Comparative Data on Caged GABA Compounds
The following table summarizes key properties of various caged GABA compounds, including this compound:
Caged Compound | IC50 (μM) | Stability | Photolysis Wavelength (nm) |
---|---|---|---|
This compound | 0.5 | Stable in dark | Near-UV / 405 |
CDNI-GABA | 0.6 | Stable in dark | Near-UV |
Bis-CNB-GABA | ≥2.5 | t1/2 = 98 days | Near-UV |
Mono-CNB-GABA | 28 | t1/2 = 138 days | Near-UV |
The data indicates that this compound exhibits favorable characteristics for experimental use, including low IC50 values and good stability under experimental conditions .
Case Study 1: Investigating Synaptic Transmission
A study utilized this compound to analyze synaptic transmission dynamics in hippocampal neurons. By applying laser photolysis, researchers were able to evoke rapid inhibitory postsynaptic currents (IPSCs), demonstrating the compound's effectiveness in studying synaptic responses under controlled conditions .
Case Study 2: Modulating Neural Circuits
In another experiment, this compound was employed to manipulate inhibitory signaling within a defined neural circuit involved in olfactory processing. The results revealed critical insights into how alterations in GABA release affect sensory processing and behavioral outcomes related to olfactory discrimination .
Mechanism of Action
DPNI-caged-GABA exerts its effects through a photoactivation mechanism. Upon exposure to light, the nitroindoline group is cleaved, releasing gamma-aminobutyric acid. The released gamma-aminobutyric acid then binds to gamma-aminobutyric acid receptors, primarily gamma-aminobutyric acid type A receptors, leading to the inhibition of neuronal activity. This mechanism allows for precise spatial and temporal control of gamma-aminobutyric acid signaling in neural tissues .
Comparison with Similar Compounds
Comparison with Similar Caged GABA Compounds
Photochemical Properties
DPNI-Caged-GABA
- Caging Group : 7-nitroindoline.
- Activation Wavelength : 405 nm (near-UV).
- Quantum Yield : 0.085, matching MNI-glutamate’s efficiency .
- Solubility : High aqueous solubility (>500 µM), critical for physiological studies .
Benzopyran-Based Caged GABA (Curten et al., 2005)
- Caging Group : 2H-1-benzopyran-2-one.
- Activation Wavelength : Near-UV (unspecified exact range).
- Quantum Yield: Not reported, but photolytic efficiency is lower than this compound due to structural bulkiness .
- Solubility : Moderate; requires organic solvents for stock solutions, limiting in vivo use .
Visible Light-Activated Caged GABA (Shembekar et al., 2005)
- Caging Group : Organic protecting group optimized for visible light.
- Activation Wavelength : Visible light (>450 nm).
- Quantum Yield: Not quantified, but visible light reduces phototoxicity compared to UV .
- Solubility : High, suitable for prolonged experiments .
Double-Caged GABA (Amatrudo et al., 2013)
- Caging Group : Dual nitroindoline groups.
- Activation Wavelength : Two-photon (740–900 nm).
- Quantum Yield : Higher effective yield due to dual uncaging steps.
- Solubility : Moderate; requires lower concentrations for effective uncaging .
Functional and Practical Comparisons
Advantages and Limitations
This compound :
Visible Light-Activated GABA :
Double-Caged GABA :
Research Findings and Case Studies
- This compound in Dendritic PSP Analysis: Used to demonstrate GABAergic postsynaptic potentials (PSPs) attenuate bidirectionally in dendrites, contrasting glutamate’s asymmetric propagation .
Double-Caged GABA in High-Throughput Screening :
Enables focal stimulation of GABA receptors with minimal off-target effects .Visible Light-Activated GABA in Developmental Studies : Facilitates long-term neuronal activity modulation without UV-induced damage .
Biological Activity
DPNI-caged-GABA (DPNI-GABA) is a synthetic compound designed to study the biological activity of GABA (gamma-aminobutyric acid) in neuronal systems. This compound is part of a class known as "caged" compounds, which are chemically modified to be inactive until exposed to light, allowing for precise temporal and spatial control of neurotransmitter release. The unique properties of DPNI-GABA make it particularly valuable for investigating GABAA receptor dynamics and synaptic transmission.
DPNI-GABA is synthesized through the attachment of a nitroindoline moiety, which serves as the caging group. This structure provides the compound with specific characteristics that enhance its utility in photolytic studies. The synthesis involves using an anionically substituted nitroindoline that reduces the affinity for GABA receptors when uncaged, thus facilitating more controlled experimental conditions .
Table 1: Comparative Properties of Caged GABA Compounds
Caged Compound | IC50 (μM) | Stability (t1/2) |
---|---|---|
DPNI-GABA | 500 | Stable in dark |
CNB-GABA | 28 | 138 days |
Bis-CNB-GABA | ≥2500 | 98 days |
RuBi-GABA | 100-300 | Stable in dark |
DPNI-GABA acts primarily on GABAA receptors, which are crucial for mediating inhibitory neurotransmission in the central nervous system. Upon photolytic uncaging, DPNI-GABA releases GABA, leading to receptor activation and subsequent neuronal inhibition. The kinetics of this process are essential for studying rapid synaptic events and understanding the dynamics of neurotransmitter release and receptor activation .
Case Studies
- Kinetic Studies : Research has demonstrated that DPNI-GABA allows for fast kinetic studies due to its favorable photolysis properties. In experiments involving laser photolysis, DPNI-GABA was shown to effectively silence neurons in situ, providing insights into neuronal behavior under controlled conditions .
- Comparison with Other Caged Compounds : Studies comparing DPNI-GABA with other caged compounds indicate its superior performance in terms of speed and reduced background activity. For instance, while bis-CNB-GABA exhibits significant antagonistic activity at GABAA receptors, DPNI-GABA shows reduced receptor affinity prior to photolysis, making it a more effective tool for precise measurements .
- Neuronal Communication : In a study exploring synaptic communication, DPNI-GABA was utilized to probe single-synapse interactions. The ability to control GABA release with high spatial resolution enabled researchers to investigate the role of GABAA receptors in synaptic integration and plasticity .
Photolytic Efficiency
The efficiency of DPNI-GABA's photolysis has been quantified through various experimental setups. The compound demonstrates rapid uncaging kinetics, with studies reporting effective neurotransmitter release within milliseconds after exposure to light. This rapid response is critical for mimicking physiological conditions during synaptic transmission studies .
Stability and Handling
DPNI-GABA exhibits stability under dark conditions, which is advantageous for experimental setups requiring prolonged handling or storage prior to use. Its stability allows researchers to prepare stock solutions without immediate degradation, ensuring reliable experimental outcomes .
Q & A
Basic Research Questions
Q. What are the key photochemical properties of DPNI-caged-GABA, and how do they influence experimental design in neural circuit studies?
this compound exhibits a quantum yield of 0.085, comparable to MNI-glutamate, enabling rapid photorelease (~millisecond timescale) under near-UV or 405 nm laser illumination . Its high water solubility (>200 µM) allows direct application in physiological buffers without organic solvents, critical for maintaining neuronal viability. Researchers should calibrate laser power (4–6 mW) and duration (1–5 ms pulses) to achieve localized GABA uncaging while minimizing phototoxicity .
Q. What protocols are recommended for preparing this compound solutions to ensure stability and reproducibility?
Dissolve this compound in distilled water or HEPES-buffered saline (pH 7.4) to a stock concentration of 10–20 mM. Aliquot and store at -20°C in light-protected vials. Pre-experiment validation via HPLC (≥98% purity) and absorbance spectroscopy (λmax = 350–405 nm) is advised to confirm compound integrity .
Q. How does this compound compare to other caged neurotransmitters (e.g., MNI-glutamate) in terms of spatiotemporal resolution?
this compound achieves comparable uncaging kinetics (~1 ms) to MNI-glutamate but requires lower UV intensities due to its nitroindoline backbone. However, its larger molecular weight (627.4 g/mol) may reduce diffusion rates in dense tissue, necessitating proximity to target receptors (<50 µm) for precise synaptic mimicry .
Advanced Research Questions
Q. What experimental strategies mitigate off-target effects during two-photon this compound uncaging in vivo?
Two-photon excitation (720–900 nm) reduces scattering and photodamage in deep tissue. Use <500 µM this compound to minimize basal receptor activation. Control experiments should include:
- Caged-compound-only controls (no light) to assess spontaneous hydrolysis.
- Light-only controls (no compound) to rule out laser-induced artifacts.
- Pharmacological blockers (e.g., gabazine) to confirm GABAA receptor specificity .
Q. How can researchers resolve contradictions in this compound efficacy across different neuronal subtypes?
Variability in GABAA receptor subunit composition (e.g., α1 vs. α5 subunits) affects binding affinity. For example:
Subtype | EC50 (µM) | Reference |
---|---|---|
α1β2γ2 | 12.3 ± 1.5 | |
α5β3γ2 | 28.7 ± 3.2 | |
Address discrepancies by: |
- Validating receptor expression via immunostaining.
- Adjusting uncaging laser power or duration to match receptor density .
Q. What computational models support the integration of this compound data into network-level simulations?
Use Hodgkin-Huxley-type models incorporating GABAA receptor kinetics (τrise = 0.5 ms, τdecay = 5–20 ms). Parameterize simulations with experimental data from Trigo et al. (2009), which quantified presynaptic GABAergic currents in interneurons using this compound .
Q. How do temperature and pH affect this compound stability in long-duration imaging experiments?
At physiological pH (7.4), spontaneous hydrolysis rates are <0.1%/hour. However, temperatures >37°C accelerate degradation (0.5%/hour at 40°C). For prolonged experiments (>2 hours), replenish the bath solution every 60 minutes and monitor pH drift with fluorescent indicators (e.g., SNARF-1) .
Q. Methodological Considerations
Q. What statistical approaches are recommended for analyzing this compound dose-response relationships?
Fit data with a four-parameter logistic equation:
where = Hill coefficient. Report 95% confidence intervals for EC50 and using bootstrap resampling (n ≥ 5 replicates) .
Q. How can this compound be combined with optogenetic tools for multiplexed neuromodulation?
Employ spectral separation:
- Use 470 nm light for ChR2 activation.
- Apply 405 nm pulses for this compound uncaging. Validate crosstalk by testing ChR2 responses under 405 nm illumination (typically <5% activation) .
Properties
IUPAC Name |
[2-[[1-(4-aminobutanoyl)-7-nitro-2,3-dihydroindol-4-yl]oxy]-3-phosphonooxypropyl] dihydrogen phosphate | |
---|---|---|
Source | PubChem | |
URL | https://pubchem.ncbi.nlm.nih.gov | |
Description | Data deposited in or computed by PubChem | |
InChI |
InChI=1S/C15H23N3O12P2/c16-6-1-2-14(19)17-7-5-11-13(4-3-12(15(11)17)18(20)21)30-10(8-28-31(22,23)24)9-29-32(25,26)27/h3-4,10H,1-2,5-9,16H2,(H2,22,23,24)(H2,25,26,27) | |
Source | PubChem | |
URL | https://pubchem.ncbi.nlm.nih.gov | |
Description | Data deposited in or computed by PubChem | |
InChI Key |
QLCHBTSDKGMLRN-UHFFFAOYSA-N | |
Source | PubChem | |
URL | https://pubchem.ncbi.nlm.nih.gov | |
Description | Data deposited in or computed by PubChem | |
Canonical SMILES |
C1CN(C2=C(C=CC(=C21)OC(COP(=O)(O)O)COP(=O)(O)O)[N+](=O)[O-])C(=O)CCCN | |
Source | PubChem | |
URL | https://pubchem.ncbi.nlm.nih.gov | |
Description | Data deposited in or computed by PubChem | |
Molecular Formula |
C15H23N3O12P2 | |
Source | PubChem | |
URL | https://pubchem.ncbi.nlm.nih.gov | |
Description | Data deposited in or computed by PubChem | |
Molecular Weight |
499.30 g/mol | |
Source | PubChem | |
URL | https://pubchem.ncbi.nlm.nih.gov | |
Description | Data deposited in or computed by PubChem | |
Retrosynthesis Analysis
AI-Powered Synthesis Planning: Our tool employs the Template_relevance Pistachio, Template_relevance Bkms_metabolic, Template_relevance Pistachio_ringbreaker, Template_relevance Reaxys, Template_relevance Reaxys_biocatalysis model, leveraging a vast database of chemical reactions to predict feasible synthetic routes.
One-Step Synthesis Focus: Specifically designed for one-step synthesis, it provides concise and direct routes for your target compounds, streamlining the synthesis process.
Accurate Predictions: Utilizing the extensive PISTACHIO, BKMS_METABOLIC, PISTACHIO_RINGBREAKER, REAXYS, REAXYS_BIOCATALYSIS database, our tool offers high-accuracy predictions, reflecting the latest in chemical research and data.
Strategy Settings
Precursor scoring | Relevance Heuristic |
---|---|
Min. plausibility | 0.01 |
Model | Template_relevance |
Template Set | Pistachio/Bkms_metabolic/Pistachio_ringbreaker/Reaxys/Reaxys_biocatalysis |
Top-N result to add to graph | 6 |
Feasible Synthetic Routes
Disclaimer and Information on In-Vitro Research Products
Please be aware that all articles and product information presented on BenchChem are intended solely for informational purposes. The products available for purchase on BenchChem are specifically designed for in-vitro studies, which are conducted outside of living organisms. In-vitro studies, derived from the Latin term "in glass," involve experiments performed in controlled laboratory settings using cells or tissues. It is important to note that these products are not categorized as medicines or drugs, and they have not received approval from the FDA for the prevention, treatment, or cure of any medical condition, ailment, or disease. We must emphasize that any form of bodily introduction of these products into humans or animals is strictly prohibited by law. It is essential to adhere to these guidelines to ensure compliance with legal and ethical standards in research and experimentation.