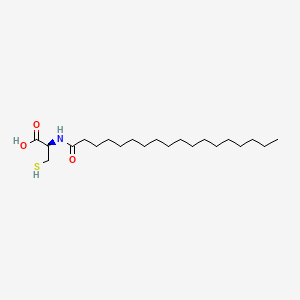
N-Stearoyl-L-cysteine
Overview
Description
N-Stearoyl-L-cysteine is a carboxylic acid derivative that has garnered attention due to its neuroprotective properties. It is an active compound in the production of various pharmaceuticals and industrial chemicals, including medications for the treatment of HIV, cancer, and stroke . This compound has also been demonstrated to have protective effects on brain cells following cerebral ischemia by reducing oxidative stress and inhibiting lipid peroxidation .
Mechanism of Action
Target of Action
N-Stearoyl-L-cysteine is a carboxylic acid that has been shown to have neuroprotective properties . It is an active compound in the production of a number of pharmaceuticals and industrial chemicals It is known to interact with biological targets such as proteins .
Mode of Action
It is known that this compound has neuroprotective effects, which could be due to its interaction with its targets and any resulting changes
Biochemical Pathways
This compound may affect various biochemical pathways. For instance, it has been shown to have neuroprotective effects in animal models of brain injury by reducing oxidative stress and inhibiting lipid peroxidation . This suggests that it may influence pathways related to oxidative stress and lipid metabolism.
Result of Action
This compound has been demonstrated to have neuroprotective effects in animal models of brain injury by reducing oxidative stress and inhibiting lipid peroxidation . This compound has also been shown to have a protective effect on brain cells following cerebral ischemia (a shortage of blood) . These results suggest that this compound can have significant molecular and cellular effects.
Biochemical Analysis
Biochemical Properties
N-Stearoyl-L-cysteine is part of the N-acyl amino acids (NA-AAs) class within the fatty acid amide family . It interacts with various enzymes, proteins, and other biomolecules in biochemical reactions. For instance, it is known to interact with N-acyl amino acid synthases expressed in E. coli .
Cellular Effects
It is known that N-acyl amino acids, including this compound, can influence cell function, including impacts on cell signaling pathways, gene expression, and cellular metabolism .
Molecular Mechanism
It is likely to exert its effects at the molecular level through binding interactions with biomolecules, enzyme inhibition or activation, and changes in gene expression .
Metabolic Pathways
This compound is involved in various metabolic pathways. It interacts with enzymes and cofactors, and can affect metabolic flux or metabolite levels .
Preparation Methods
Synthetic Routes and Reaction Conditions
The synthesis of N-Stearoyl-L-cysteine typically involves the acylation of L-cysteine with stearic acid or its derivatives. One common method includes the reaction of L-cysteine with stearoyl chloride in the presence of a base such as triethylamine. The reaction is carried out in an organic solvent like dichloromethane at low temperatures to prevent racemization and oxidation of the intermediate products .
Industrial Production Methods
Industrial production of this compound often employs green chemistry principles to enhance efficiency and reduce environmental impact. A novel green process involves the acylation of L-cystine followed by electrochemical reduction and electrodialysis to produce high-quality this compound. This method avoids racemization and oxidation, achieves impurity removal, and recycles electrolytes, making it suitable for large-scale production .
Chemical Reactions Analysis
Types of Reactions
N-Stearoyl-L-cysteine undergoes various chemical reactions, including:
Oxidation: The thiol group in the cysteine moiety can be oxidized to form disulfides.
Reduction: The disulfide bonds can be reduced back to thiol groups using reducing agents like dithiothreitol.
Substitution: The carboxyl group can participate in esterification or amidation reactions.
Common Reagents and Conditions
Oxidation: Hydrogen peroxide or atmospheric oxygen can oxidize the thiol group.
Reduction: Dithiothreitol or tris(2-carboxyethyl)phosphine are commonly used reducing agents.
Substitution: Esterification can be carried out using alcohols in the presence of acid catalysts, while amidation can be achieved using amines and coupling agents like carbodiimides.
Major Products
Oxidation: Formation of disulfides.
Reduction: Regeneration of thiol groups.
Substitution: Formation of esters or amides depending on the reagents used.
Scientific Research Applications
N-Stearoyl-L-cysteine has a wide range of scientific research applications:
Chemistry: Used as a building block in the synthesis of more complex molecules.
Biology: Studied for its role in cellular redox reactions and as a component of antioxidant systems.
Industry: Utilized in the production of pharmaceuticals and as an additive in various industrial processes.
Comparison with Similar Compounds
N-Stearoyl-L-cysteine is part of a broader class of N-acyl amino acids, which includes compounds like N-stearoyl-L-tyrosine and N-linoleoyl-L-tyrosine. These compounds share similar neuroprotective properties but differ in their specific amino acid components and fatty acid chains . This compound is unique due to its cysteine moiety, which contributes to its potent antioxidant activity and ability to modulate redox reactions .
List of Similar Compounds
- N-stearoyl-L-tyrosine
- N-linoleoyl-L-tyrosine
- N-palmitoyl-L-tyrosine
- N-stearoyl-L-serine
- N-stearoyl-L-threonine
Properties
IUPAC Name |
(2R)-2-(octadecanoylamino)-3-sulfanylpropanoic acid | |
---|---|---|
Source | PubChem | |
URL | https://pubchem.ncbi.nlm.nih.gov | |
Description | Data deposited in or computed by PubChem | |
InChI |
InChI=1S/C21H41NO3S/c1-2-3-4-5-6-7-8-9-10-11-12-13-14-15-16-17-20(23)22-19(18-26)21(24)25/h19,26H,2-18H2,1H3,(H,22,23)(H,24,25)/t19-/m0/s1 | |
Source | PubChem | |
URL | https://pubchem.ncbi.nlm.nih.gov | |
Description | Data deposited in or computed by PubChem | |
InChI Key |
VJUDNRUDRQLCDX-IBGZPJMESA-N | |
Source | PubChem | |
URL | https://pubchem.ncbi.nlm.nih.gov | |
Description | Data deposited in or computed by PubChem | |
Canonical SMILES |
CCCCCCCCCCCCCCCCCC(=O)NC(CS)C(=O)O | |
Source | PubChem | |
URL | https://pubchem.ncbi.nlm.nih.gov | |
Description | Data deposited in or computed by PubChem | |
Isomeric SMILES |
CCCCCCCCCCCCCCCCCC(=O)N[C@@H](CS)C(=O)O | |
Source | PubChem | |
URL | https://pubchem.ncbi.nlm.nih.gov | |
Description | Data deposited in or computed by PubChem | |
Molecular Formula |
C21H41NO3S | |
Source | PubChem | |
URL | https://pubchem.ncbi.nlm.nih.gov | |
Description | Data deposited in or computed by PubChem | |
Molecular Weight |
387.6 g/mol | |
Source | PubChem | |
URL | https://pubchem.ncbi.nlm.nih.gov | |
Description | Data deposited in or computed by PubChem | |
Retrosynthesis Analysis
AI-Powered Synthesis Planning: Our tool employs the Template_relevance Pistachio, Template_relevance Bkms_metabolic, Template_relevance Pistachio_ringbreaker, Template_relevance Reaxys, Template_relevance Reaxys_biocatalysis model, leveraging a vast database of chemical reactions to predict feasible synthetic routes.
One-Step Synthesis Focus: Specifically designed for one-step synthesis, it provides concise and direct routes for your target compounds, streamlining the synthesis process.
Accurate Predictions: Utilizing the extensive PISTACHIO, BKMS_METABOLIC, PISTACHIO_RINGBREAKER, REAXYS, REAXYS_BIOCATALYSIS database, our tool offers high-accuracy predictions, reflecting the latest in chemical research and data.
Strategy Settings
Precursor scoring | Relevance Heuristic |
---|---|
Min. plausibility | 0.01 |
Model | Template_relevance |
Template Set | Pistachio/Bkms_metabolic/Pistachio_ringbreaker/Reaxys/Reaxys_biocatalysis |
Top-N result to add to graph | 6 |
Feasible Synthetic Routes
Disclaimer and Information on In-Vitro Research Products
Please be aware that all articles and product information presented on BenchChem are intended solely for informational purposes. The products available for purchase on BenchChem are specifically designed for in-vitro studies, which are conducted outside of living organisms. In-vitro studies, derived from the Latin term "in glass," involve experiments performed in controlled laboratory settings using cells or tissues. It is important to note that these products are not categorized as medicines or drugs, and they have not received approval from the FDA for the prevention, treatment, or cure of any medical condition, ailment, or disease. We must emphasize that any form of bodily introduction of these products into humans or animals is strictly prohibited by law. It is essential to adhere to these guidelines to ensure compliance with legal and ethical standards in research and experimentation.