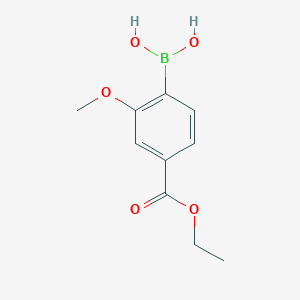
4-Ethoxycarbonyl-2-methoxyphenylboronic acid
Overview
Description
4-Ethoxycarbonyl-2-methoxyphenylboronic acid is a boronic acid derivative featuring a phenyl ring substituted with an ethoxycarbonyl (-COOEt) group at the para position and a methoxy (-OCH₃) group at the ortho position. This compound is structurally tailored for applications in cross-coupling reactions, such as Suzuki-Miyaura couplings, where boronic acids serve as key intermediates in synthesizing biaryl structures for pharmaceuticals and agrochemicals.
Preparation Methods
Synthetic Routes and Reaction Conditions
The synthesis of 4-Ethoxycarbonyl-2-methoxyphenylboronic acid typically involves the borylation of the corresponding aryl halide. One common method is the palladium-catalyzed borylation of 4-ethoxycarbonyl-2-methoxyphenyl halide using bis(pinacolato)diboron in the presence of a base such as potassium acetate. The reaction is carried out under an inert atmosphere at elevated temperatures .
Industrial Production Methods
Industrial production methods for this compound are similar to laboratory-scale synthesis but are optimized for larger scales. This includes the use of continuous flow reactors to enhance reaction efficiency and yield. The purification process often involves crystallization or chromatography to obtain the desired purity .
Chemical Reactions Analysis
Types of Reactions
4-Ethoxycarbonyl-2-methoxyphenylboronic acid undergoes various chemical reactions, including:
Suzuki-Miyaura Coupling: This is the most common reaction, where it reacts with aryl or vinyl halides in the presence of a palladium catalyst to form biaryl or substituted alkenes.
Oxidative Hydroxylation: This reaction converts the boronic acid group to a hydroxyl group, forming phenols.
Homolytic Aromatic Substitution: This reaction involves the substitution of the boronic acid group with other functional groups under radical conditions.
Common Reagents and Conditions
Palladium Catalysts: Used in Suzuki-Miyaura coupling reactions.
Bases: Such as potassium carbonate or sodium hydroxide, used to deprotonate the boronic acid group.
Oxidizing Agents: Such as hydrogen peroxide, used in oxidative hydroxylation reactions.
Major Products
Biaryls and Substituted Alkenes: Formed from Suzuki-Miyaura coupling.
Phenols: Formed from oxidative hydroxylation.
Scientific Research Applications
Organic Synthesis
Cross-Coupling Reactions
4-Ethoxycarbonyl-2-methoxyphenylboronic acid is widely utilized in Suzuki-Miyaura coupling reactions, which are essential for forming carbon-carbon bonds. This reaction is crucial in the synthesis of complex organic molecules, including pharmaceuticals and agrochemicals. The boronic acid acts as a nucleophile, coupling with aryl halides under palladium catalysis to yield biaryl compounds.
Table 1: Reaction Conditions for Suzuki Coupling
Reactants | Catalyst | Solvent | Temperature | Yield (%) |
---|---|---|---|---|
Aryl Halide + Boronic Acid | Pd(PPh₃)₄ | Toluene | 80 °C | 85-90 |
Aryl Halide + Boronic Acid | Pd(OAc)₂ | DMF | 100 °C | 75-80 |
Electrochemical Sensing
This compound has been developed as an electrochemical redox probe for the selective detection of hydrogen peroxide (H₂O₂) in biological systems. This application is particularly valuable in monitoring cellular processes and oxidative stress.
Case Study: Detection of H₂O₂ in Live Cells
A study demonstrated that the compound could be used to create a sensitive electrochemical sensor that detects H₂O₂ levels in live Caco-2 and MCF-7 cells. The probe undergoes a chemical transformation upon interaction with H₂O₂, allowing for real-time monitoring of oxidative stress within cells. This capability is crucial for understanding various pathological conditions and cellular responses to oxidative damage.
Material Science
Graphene Synthesis
In material science, this compound is employed in the chemical vapor deposition (CVD) process for synthesizing doped graphene. By incorporating this compound during graphene growth, researchers can achieve phosphorus or boron doping, which enhances the electronic properties of graphene.
Table 2: Properties of Doped Graphene
Doping Element | Electrical Conductivity (S/m) | Band Gap (eV) |
---|---|---|
Phosphorus | 1.5 × 10^4 | 0.3 |
Boron | 1.2 × 10^5 | 0.1 |
Medicinal Chemistry
This compound serves as an important intermediate in the synthesis of various biologically active compounds, including potential pharmaceuticals targeting cancer and other diseases. Its ability to form stable complexes with other organic molecules makes it a valuable component in drug development.
Example Applications:
- Synthesis of inhibitors targeting specific enzymes involved in cancer progression.
- Development of herbicides through its derivatives, enhancing agricultural productivity.
Mechanism of Action
The primary mechanism of action for 4-Ethoxycarbonyl-2-methoxyphenylboronic acid is through its role in Suzuki-Miyaura coupling reactions. The process involves the following steps:
Oxidative Addition: The palladium catalyst forms a complex with the aryl halide, resulting in the oxidative addition of the aryl group to the palladium.
Transmetalation: The boronic acid transfers its aryl group to the palladium complex.
Reductive Elimination: The palladium complex undergoes reductive elimination to form the biaryl product and regenerate the palladium catalyst.
Comparison with Similar Compounds
Comparative Analysis with Structurally Similar Compounds
The reactivity, stability, and applications of 4-ethoxycarbonyl-2-methoxyphenylboronic acid are influenced by its substituents. Below is a comparative analysis with key analogs:
Table 1: Comparison of this compound with Analogous Boronic Acids
Key Observations:
Electronic Effects: The ethoxycarbonyl (-COOEt) group is a moderate electron-withdrawing substituent, which polarizes the phenyl ring and enhances the boronic acid's reactivity in cross-coupling reactions compared to purely electron-donating groups (e.g., -OCH₃) .
Steric Considerations :
- Ortho-substituted methoxy groups (as in the target compound) introduce steric hindrance, which may slow reaction rates but improve regioselectivity in coupling reactions compared to para-substituted analogs .
Solubility and Stability: Ester groups (e.g., -COOEt or -COOMe) improve solubility in polar aprotic solvents (e.g., DMF, acetonitrile) compared to non-functionalized boronic acids. However, they may hydrolyze under strongly acidic or basic conditions . Compounds with chloro substituents (e.g., [603122-80-1]) exhibit enhanced stability under acidic conditions, making them suitable for reactions requiring low pH .
Biological Activity
4-Ethoxycarbonyl-2-methoxyphenylboronic acid is a boronic acid derivative that has garnered interest in medicinal chemistry due to its potential biological activities. This compound, characterized by its unique structural features, is being investigated for various therapeutic applications, particularly in cancer treatment and as a biochemical tool in research.
Chemical Structure and Properties
The chemical formula of this compound is C12H15B O4, and it contains a boron atom bonded to a phenyl group with ethoxycarbonyl and methoxy substituents. The presence of these functional groups influences its reactivity and biological interactions.
The mechanism of action for this compound involves its ability to interact with biological targets, such as enzymes and receptors. Boronic acids are known to form reversible covalent bonds with diols, which can inhibit enzyme activity or modulate receptor functions. This property is particularly relevant in the context of cancer therapy, where targeting specific pathways can lead to apoptosis in malignant cells.
Biological Activities
Research has indicated several biological activities associated with this compound:
- Anticancer Activity : Similar boronic acids have shown promise as proteasome inhibitors, which are crucial in cancer treatment. For instance, compounds like bortezomib have been effective against multiple myeloma by inhibiting the proteasome's function, leading to apoptosis in cancer cells .
- Antimicrobial Properties : Some studies suggest that boronic acids exhibit antimicrobial activity, potentially making them useful in treating infections caused by resistant bacteria .
- Enzyme Inhibition : The ability of boronic acids to inhibit specific enzymes involved in metabolic pathways could be leveraged for therapeutic purposes, particularly in metabolic disorders or cancer .
Case Studies
A review of recent literature highlights various studies involving boronic acids:
- Study on Bortezomib : Bortezomib, a well-known boronic acid derivative, was evaluated for its efficacy in combination therapies for aggressive lymphomas. The study found that combining bortezomib with other agents improved patient outcomes significantly .
- Research on Antimicrobial Activity : A study explored the antibacterial properties of different boronic acids, including derivatives similar to this compound. Results indicated significant inhibition of bacterial growth, suggesting potential applications in antibiotic development .
Data Table: Comparison of Biological Activities
Q & A
Basic Research Questions
Q. What are the optimal synthetic routes for 4-ethoxycarbonyl-2-methoxyphenylboronic acid, and how can reaction conditions be tailored to improve yield?
Methodological Answer: The compound can be synthesized via Suzuki-Miyaura coupling or direct boronation of substituted benzene precursors. Key parameters include:
- Catalyst selection : Pd(PPh₃)₄ or PdCl₂(dppf) for cross-coupling efficiency .
- Temperature control : Maintaining 80–100°C during boronation prevents decomposition of sensitive intermediates .
- Solvent optimization : Tetrahydrofuran (THF) or dioxane enhances boronic acid stability during reactions .
- Purification : Column chromatography (silica gel, ethyl acetate/hexane) resolves ester and boronic acid byproducts .
Q. What safety precautions are critical when handling this compound in laboratory settings?
Methodological Answer:
- PPE : Wear nitrile gloves, chemical-resistant lab coats, and safety goggles to avoid skin/eye irritation (Category 2A hazard) .
- Ventilation : Use fume hoods to minimize inhalation of dust or vapors .
- Spill management : Neutralize spills with inert adsorbents (e.g., vermiculite) and avoid aqueous rinsing to prevent environmental contamination .
- Storage : Keep in airtight containers at 0–6°C under inert gas (N₂/Ar) to prevent oxidation .
Q. How can researchers assess the stability of this compound under varying experimental conditions?
Methodological Answer:
- Thermal stability : Conduct differential scanning calorimetry (DSC) to identify decomposition temperatures (>148°C observed in analogs) .
- Hydrolytic stability : Monitor boronic acid integrity via ¹¹B NMR in aqueous/organic solvent mixtures (e.g., pH 7–9 buffers) .
- Oxidative sensitivity : Test compatibility with common oxidizers (e.g., H₂O₂) using FT-IR to detect B-O bond cleavage .
Q. What analytical techniques are most effective for characterizing this compound and its intermediates?
Methodological Answer:
- Structural confirmation : ¹H/¹³C NMR (δ ~7.5–8.0 ppm for aromatic protons) and HRMS (expected [M+H]⁺ ~237.08) .
- Purity analysis : Reverse-phase HPLC (C18 column, acetonitrile/water gradient) detects residual esters or boroxines .
- Crystallinity : X-ray diffraction (analogs show monoclinic crystal systems) .
Advanced Research Questions
Q. How can contradictory data on the reactivity of this compound in cross-coupling reactions be resolved?
Methodological Answer:
- Mechanistic studies : Use kinetic isotope effects (KIE) or DFT calculations to identify rate-limiting steps (e.g., transmetallation vs. reductive elimination) .
- Byproduct profiling : LC-MS/MS to detect deborylation products or ester hydrolysis under basic conditions .
- Catalyst screening : Compare Pd-, Ni-, or Cu-based catalysts for substrate-specific efficiency .
Q. What strategies minimize byproduct formation during the synthesis of this compound derivatives?
Methodological Answer:
- Protecting groups : Temporarily mask the boronic acid with pinacol ester to prevent protodeboronation .
- Microwave-assisted synthesis : Reduces reaction time and side reactions (e.g., 10 min at 120°C vs. 24 hr conventional) .
- Additives : Use K₂CO₃ or CsF to stabilize boronate intermediates in Suzuki couplings .
Q. How does the electron-withdrawing ethoxycarbonyl group influence the reactivity of the boronic acid moiety in organometallic reactions?
Methodological Answer:
- Electrostatic potential maps : Computational modeling (e.g., Gaussian) shows increased Lewis acidity at boron, enhancing transmetallation rates .
- Comparative kinetics : Measure reaction rates vs. non-substituted analogs (e.g., 2-methoxyphenylboronic acid) under identical conditions .
- Substituent effects : Hammett plots correlate σₚ values of substituents with catalytic turnover .
Q. What methodologies evaluate the environmental impact of this compound in laboratory waste streams?
Methodological Answer:
- Ecotoxicology assays : Use Daphnia magna or Vibrio fischeri to assess acute toxicity (EC₅₀ values) .
- Degradation studies : UV/H₂O₂ treatment or biodegradation screening (OECD 301F) to quantify persistence .
- Waste neutralization : Incineration at >800°C with alkaline scrubbers to capture boron oxides .
Q. How can solvent polarity and solubility parameters be optimized for this compound in aqueous-organic biphasic systems?
Methodological Answer:
- Solubility screening : Use shake-flask method with logP calculations (predicted ~1.5–2.0) .
- Phase-transfer catalysts : Employ tetrabutylammonium bromide (TBAB) to enhance solubility in polar solvents .
- Co-solvent blends : THF/water (4:1 v/v) balances reactivity and boronic acid stability .
Q. What are the challenges in crystallizing this compound, and how can they be addressed?
Methodological Answer:
- Crystallization solvents : Slow evaporation from ethyl acetate/hexane (3:7) minimizes ester hydrolysis .
- Polymorph screening : High-throughput crystallization (e.g., Crystal16) identifies stable forms .
- Crystal engineering : Co-crystallization with triphenylphosphine oxide improves lattice stability .
Properties
IUPAC Name |
(4-ethoxycarbonyl-2-methoxyphenyl)boronic acid | |
---|---|---|
Details | Computed by LexiChem 2.6.6 (PubChem release 2019.06.18) | |
Source | PubChem | |
URL | https://pubchem.ncbi.nlm.nih.gov | |
Description | Data deposited in or computed by PubChem | |
InChI |
InChI=1S/C10H13BO5/c1-3-16-10(12)7-4-5-8(11(13)14)9(6-7)15-2/h4-6,13-14H,3H2,1-2H3 | |
Details | Computed by InChI 1.0.5 (PubChem release 2019.06.18) | |
Source | PubChem | |
URL | https://pubchem.ncbi.nlm.nih.gov | |
Description | Data deposited in or computed by PubChem | |
InChI Key |
BGZDEQUZDOOMMD-UHFFFAOYSA-N | |
Details | Computed by InChI 1.0.5 (PubChem release 2019.06.18) | |
Source | PubChem | |
URL | https://pubchem.ncbi.nlm.nih.gov | |
Description | Data deposited in or computed by PubChem | |
Canonical SMILES |
B(C1=C(C=C(C=C1)C(=O)OCC)OC)(O)O | |
Details | Computed by OEChem 2.1.5 (PubChem release 2019.06.18) | |
Source | PubChem | |
URL | https://pubchem.ncbi.nlm.nih.gov | |
Description | Data deposited in or computed by PubChem | |
Molecular Formula |
C10H13BO5 | |
Details | Computed by PubChem 2.1 (PubChem release 2019.06.18) | |
Source | PubChem | |
URL | https://pubchem.ncbi.nlm.nih.gov | |
Description | Data deposited in or computed by PubChem | |
Molecular Weight |
224.02 g/mol | |
Details | Computed by PubChem 2.1 (PubChem release 2021.05.07) | |
Source | PubChem | |
URL | https://pubchem.ncbi.nlm.nih.gov | |
Description | Data deposited in or computed by PubChem | |
Synthesis routes and methods
Procedure details
Retrosynthesis Analysis
AI-Powered Synthesis Planning: Our tool employs the Template_relevance Pistachio, Template_relevance Bkms_metabolic, Template_relevance Pistachio_ringbreaker, Template_relevance Reaxys, Template_relevance Reaxys_biocatalysis model, leveraging a vast database of chemical reactions to predict feasible synthetic routes.
One-Step Synthesis Focus: Specifically designed for one-step synthesis, it provides concise and direct routes for your target compounds, streamlining the synthesis process.
Accurate Predictions: Utilizing the extensive PISTACHIO, BKMS_METABOLIC, PISTACHIO_RINGBREAKER, REAXYS, REAXYS_BIOCATALYSIS database, our tool offers high-accuracy predictions, reflecting the latest in chemical research and data.
Strategy Settings
Precursor scoring | Relevance Heuristic |
---|---|
Min. plausibility | 0.01 |
Model | Template_relevance |
Template Set | Pistachio/Bkms_metabolic/Pistachio_ringbreaker/Reaxys/Reaxys_biocatalysis |
Top-N result to add to graph | 6 |
Feasible Synthetic Routes
Disclaimer and Information on In-Vitro Research Products
Please be aware that all articles and product information presented on BenchChem are intended solely for informational purposes. The products available for purchase on BenchChem are specifically designed for in-vitro studies, which are conducted outside of living organisms. In-vitro studies, derived from the Latin term "in glass," involve experiments performed in controlled laboratory settings using cells or tissues. It is important to note that these products are not categorized as medicines or drugs, and they have not received approval from the FDA for the prevention, treatment, or cure of any medical condition, ailment, or disease. We must emphasize that any form of bodily introduction of these products into humans or animals is strictly prohibited by law. It is essential to adhere to these guidelines to ensure compliance with legal and ethical standards in research and experimentation.