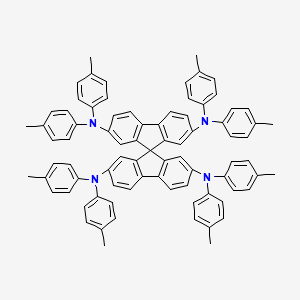
2,2',7,7'-Tetrakis(di-p-tolylamino)spiro-9,9'-bifluorene
- Click on QUICK INQUIRY to receive a quote from our team of experts.
- With the quality product at a COMPETITIVE price, you can focus more on your research.
Overview
Description
“2,2’,7,7’-Tetrakis(di-p-tolylamino)spiro-9,9’-bifluorene”, also known as Spiro-TTB, has a spirofluorene core with four attached ditolylamine at the 2 and 7 positions of spirofluorene . It is electron-rich and commonly used as a hole transport material (HTM) in OLED, OPV, and perovskite solar cells .
Molecular Structure Analysis
Spiro-TTB has a complex molecular structure. The replacement of two 4,4’-dimeoxyl-diphenylamine at the 2’ and 7’ positions of conventional spiro-OMeTAD with two tert-butyl in spiro-027 results in a greatly simplified molecular structure .Physical and Chemical Properties Analysis
Spiro-TTB has a molecular weight of 1097.43 g/mol and a predicted density of 1.28±0.1 g/cm3 . Its glass transition temperature (Tg) is 146 °C .Scientific Research Applications
Organic Light-Emitting Devices (OLEDs)
A series of fluorinated 9,9′-spirobifluorene derivatives, including those similar to 2,2',7,7'-Tetrakis(di-p-tolylamino)spiro-9,9'-bifluorene, have been synthesized for use in OLEDs. These derivatives demonstrate tunable photophysical properties, energy levels, and thermal stabilities, making them suitable for OLED applications. For instance, a deep blue OLED using a spirobifluorene derivative exhibited exceptional performance with high luminance and efficiency (Li et al., 2013).
Hole Transport Materials in Solar Cells
Spiro-OMeTAD, a molecule structurally related to this compound, is a common hole transport material in perovskite solar cells (PSCs). It is valued for its effective photovoltaic performance but has limitations like low conductivity, necessitating dopants and oxidative processes for enhancement. Research in this area focuses on improving spiro-OMeTAD's electrical properties and identifying efficient alternatives (Ouedraogo et al., 2022).
Excitonic Solar Cells
In solid-state excitonic solar cells, spiro-linked molecules, including those structurally similar to this compound, act as secondary absorbers. These molecules blend with hole-transporting materials and contribute to an extended spectral response, increasing the efficiency and current of the solar cells (Driscoll et al., 2010).
Theoretical Design for Solar Cells
Spirobifluorene derivatives have been designed and simulated for use in organic-inorganic hybrid perovskite solar cells. These derivatives are studied for alignment with perovskite materials, aiming for ideal solar cell efficiencies. The focus is on modifying the structural template to optimize absorption spectrum and efficiency (Zhang et al., 2019).
Mechanism of Action
Target of Action
The primary target of 2,2’,7,7’-Tetrakis(di-p-tolylamino)spiro-9,9’-bifluorene, also known as Spiro-TTB, is to serve as a hole transport material (HTM) in optoelectronic devices, particularly in perovskite solar cells (PSCs) . The role of HTMs is to facilitate the movement of positive charges or ‘holes’ from the active layer of the device to the electrode.
Mode of Action
Spiro-TTB interacts with its targets by providing a pathway for the transport of holes. It is an electron-rich compound, which means it has a high capacity to accept and transport positive charges . This property is crucial in devices like PSCs, where efficient charge transport is needed to achieve high power conversion efficiency .
Biochemical Pathways
In the context of PSCs, the key pathway affected by Spiro-TTB is the charge transport pathway. When light hits the perovskite layer of a PSC, it excites electrons, creating electron-hole pairs. Spiro-TTB facilitates the movement of these holes towards the electrode, preventing recombination and thus enhancing the efficiency of the solar cell .
Pharmacokinetics
While the term pharmacokinetics is typically used in the context of drug metabolism, in the case of Spiro-TTB, we can discuss its properties in terms of stability and mobility. Spiro-TTB exhibits good thermal stability and enhanced hole mobility, which are crucial for its function as an HTM .
Result of Action
The primary result of Spiro-TTB’s action is the efficient transport of holes in PSCs, which leads to high power conversion efficiency. Devices based on Spiro-TTB have demonstrated power conversion efficiencies exceeding 16%, which is higher than devices based on similar materials .
Action Environment
The performance of Spiro-TTB can be influenced by various environmental factors. For instance, the efficiency of hole transport can be affected by the morphological properties of the perovskite thin films in the PSCs . Additionally, the synthesis conditions of Spiro-TTB can also impact its performance .
Future Directions
Biochemical Analysis
Biochemical Properties
2,2’,7,7’-Tetrakis(di-p-tolylamino)spiro-9,9’-bifluorene is primarily used as a hole transport material (HTM) in perovskite solar cells (PSCs) . It is electron-rich and its four substituted arylamine moieties stabilize positively charged cationic states via mesomeric effects .
Molecular Mechanism
The molecular mechanism of 2,2’,7,7’-Tetrakis(di-p-tolylamino)spiro-9,9’-bifluorene involves its role as a hole transport material. It facilitates efficient charge transport from the anode to the emitting layers of the optoelectronic structure .
Temporal Effects in Laboratory Settings
In laboratory settings, 2,2’,7,7’-Tetrakis(di-p-tolylamino)spiro-9,9’-bifluorene demonstrates high thermal stability . Its hole mobility remains low, which may result in severe charge recombination .
Dosage Effects in Animal Models
As of now, there is no available data on the effects of 2,2’,7,7’-Tetrakis(di-p-tolylamino)spiro-9,9’-bifluorene in animal models as it is primarily used in optoelectronic applications .
Transport and Distribution
In optoelectronic devices, it is known to facilitate efficient charge transport .
Subcellular Localization
The subcellular localization of 2,2’,7,7’-Tetrakis(di-p-tolylamino)spiro-9,9’-bifluorene is not applicable as it is not typically used in biological contexts .
Properties
IUPAC Name |
2-N,2-N,2-N',2-N',7-N,7-N,7-N',7-N'-octakis(4-methylphenyl)-9,9'-spirobi[fluorene]-2,2',7,7'-tetramine |
Source
|
---|---|---|
Details | Computed by Lexichem TK 2.7.0 (PubChem release 2021.05.07) | |
Source | PubChem | |
URL | https://pubchem.ncbi.nlm.nih.gov | |
Description | Data deposited in or computed by PubChem | |
InChI |
InChI=1S/C81H68N4/c1-53-9-25-61(26-10-53)82(62-27-11-54(2)12-28-62)69-41-45-73-74-46-42-70(83(63-29-13-55(3)14-30-63)64-31-15-56(4)16-32-64)50-78(74)81(77(73)49-69)79-51-71(84(65-33-17-57(5)18-34-65)66-35-19-58(6)20-36-66)43-47-75(79)76-48-44-72(52-80(76)81)85(67-37-21-59(7)22-38-67)68-39-23-60(8)24-40-68/h9-52H,1-8H3 |
Source
|
Details | Computed by InChI 1.0.6 (PubChem release 2021.05.07) | |
Source | PubChem | |
URL | https://pubchem.ncbi.nlm.nih.gov | |
Description | Data deposited in or computed by PubChem | |
InChI Key |
VMPLMOXGWULJTH-UHFFFAOYSA-N |
Source
|
Details | Computed by InChI 1.0.6 (PubChem release 2021.05.07) | |
Source | PubChem | |
URL | https://pubchem.ncbi.nlm.nih.gov | |
Description | Data deposited in or computed by PubChem | |
Canonical SMILES |
CC1=CC=C(C=C1)N(C2=CC=C(C=C2)C)C3=CC4=C(C=C3)C5=C(C46C7=C(C=CC(=C7)N(C8=CC=C(C=C8)C)C9=CC=C(C=C9)C)C1=C6C=C(C=C1)N(C1=CC=C(C=C1)C)C1=CC=C(C=C1)C)C=C(C=C5)N(C1=CC=C(C=C1)C)C1=CC=C(C=C1)C |
Source
|
Details | Computed by OEChem 2.3.0 (PubChem release 2021.05.07) | |
Source | PubChem | |
URL | https://pubchem.ncbi.nlm.nih.gov | |
Description | Data deposited in or computed by PubChem | |
Molecular Formula |
C81H68N4 |
Source
|
Details | Computed by PubChem 2.1 (PubChem release 2021.05.07) | |
Source | PubChem | |
URL | https://pubchem.ncbi.nlm.nih.gov | |
Description | Data deposited in or computed by PubChem | |
Molecular Weight |
1097.4 g/mol |
Source
|
Details | Computed by PubChem 2.1 (PubChem release 2021.05.07) | |
Source | PubChem | |
URL | https://pubchem.ncbi.nlm.nih.gov | |
Description | Data deposited in or computed by PubChem | |
Disclaimer and Information on In-Vitro Research Products
Please be aware that all articles and product information presented on BenchChem are intended solely for informational purposes. The products available for purchase on BenchChem are specifically designed for in-vitro studies, which are conducted outside of living organisms. In-vitro studies, derived from the Latin term "in glass," involve experiments performed in controlled laboratory settings using cells or tissues. It is important to note that these products are not categorized as medicines or drugs, and they have not received approval from the FDA for the prevention, treatment, or cure of any medical condition, ailment, or disease. We must emphasize that any form of bodily introduction of these products into humans or animals is strictly prohibited by law. It is essential to adhere to these guidelines to ensure compliance with legal and ethical standards in research and experimentation.