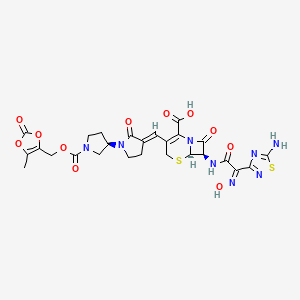
Ceftobiprole medocaril
- Click on QUICK INQUIRY to receive a quote from our team of experts.
- With the quality product at a COMPETITIVE price, you can focus more on your research.
Overview
Description
- It is marketed under the brand names Zevtera and Mabelio .
- Like other cephalosporins, ceftobiprole inhibits bacterial cell wall synthesis by binding to penicillin-binding proteins (PBPs).
Ceftobiprole Medocaril: is an injectable cephalosporin antibiotic used to treat hospital-acquired pneumonia (excluding ventilator-associated pneumonia) and community-acquired pneumonia.
Mechanism of Action
Target of Action
Ceftobiprole medocaril, identified by the Unique Ingredient Identifier (UNII) YXV28V1B07 , is a prodrug of ceftobiprole . Ceftobiprole is a fifth-generation semisynthetic cephalosporin antibacterial . The primary targets of this compound are the penicillin-binding proteins (PBPs), which play a crucial role in bacterial cell wall synthesis .
Mode of Action
Ceftobiprole, the active moiety of this compound, exhibits its bactericidal activity by inhibiting bacterial cell wall synthesis . This activity is mediated through binding to essential PBPs and inhibiting their transpeptidase activity, which is essential for the synthesis of the bacterial cell wall .
Biochemical Analysis
Biochemical Properties
Ceftobiprole medocaril plays a crucial role in biochemical reactions by inhibiting bacterial cell wall synthesis. It interacts with penicillin-binding proteins (PBPs), which are essential for the cross-linking of peptidoglycan chains in the bacterial cell wall. By binding to these proteins, this compound prevents the formation of a functional cell wall, leading to bacterial cell death . The compound’s interaction with PBPs is highly specific, making it effective against a wide range of bacterial pathogens .
Cellular Effects
This compound exerts significant effects on various types of cells and cellular processes. In bacterial cells, it disrupts cell wall synthesis, leading to cell lysis and death. This disruption affects cell signaling pathways, gene expression, and cellular metabolism. The compound’s ability to bind to PBPs inhibits the normal function of these proteins, resulting in the accumulation of peptidoglycan precursors and the activation of autolytic enzymes, which further degrade the cell wall . In mammalian cells, this compound has minimal direct effects, as it specifically targets bacterial enzymes .
Molecular Mechanism
The molecular mechanism of this compound involves its conversion to the active form, ceftobiprole, which then binds to PBPs. This binding inhibits the transpeptidase activity of PBPs, preventing the cross-linking of peptidoglycan chains and leading to cell wall disruption . Additionally, this compound has been shown to interact with the spike proteins of SARS-CoV and SARS-CoV-2, suggesting potential antiviral properties . The compound’s ability to inhibit bacterial cell wall synthesis and potentially interfere with viral proteins highlights its broad-spectrum activity .
Temporal Effects in Laboratory Settings
In laboratory settings, the effects of this compound have been observed to change over time. The compound is stable under standard storage conditions, but its activity can degrade over extended periods. In vitro studies have shown that this compound maintains its antibacterial activity for several hours after administration, with a gradual decrease in efficacy over time . Long-term studies in vivo have demonstrated that repeated administration of this compound can lead to the development of bacterial resistance, highlighting the importance of appropriate dosing and duration of treatment .
Dosage Effects in Animal Models
The effects of this compound vary with different dosages in animal models. At therapeutic doses, the compound effectively treats bacterial infections without significant adverse effects. At higher doses, this compound can cause toxicity, including nephrotoxicity and hepatotoxicity . Studies in animal models have shown that the compound’s efficacy is dose-dependent, with higher doses leading to increased antibacterial activity but also a higher risk of adverse effects . It is crucial to determine the optimal dosage to balance efficacy and safety in clinical use .
Metabolic Pathways
This compound is metabolized in the liver, where it is converted to its active form, ceftobiprole. The metabolic pathways involved include hydrolysis and oxidation reactions, mediated by liver enzymes . The compound’s metabolites are primarily excreted through the kidneys, with a small fraction eliminated via the bile . The interaction of this compound with liver enzymes can affect metabolic flux and alter the levels of various metabolites, influencing its overall pharmacokinetics and pharmacodynamics .
Transport and Distribution
Within cells and tissues, this compound is transported and distributed via passive diffusion and active transport mechanisms. The compound interacts with various transporters and binding proteins, which facilitate its uptake and distribution . This compound is widely distributed in body tissues, including the lungs, skin, and kidneys, where it exerts its antibacterial effects . The compound’s distribution is influenced by factors such as tissue perfusion, binding affinity, and the presence of transport proteins .
Subcellular Localization
This compound is primarily localized in the bacterial cell wall, where it exerts its antibacterial activity. The compound’s targeting signals and post-translational modifications direct it to the cell wall, allowing it to interact with PBPs and inhibit cell wall synthesis . In mammalian cells, this compound does not accumulate significantly, as it is rapidly metabolized and excreted . The specific localization of this compound in bacterial cells is crucial for its efficacy and selectivity as an antibiotic .
Preparation Methods
Industrial Production: Industrial-scale production methods involve optimization of synthetic steps, purification, and formulation for intravenous administration.
Chemical Reactions Analysis
Reactivity: Ceftobiprole undergoes various reactions, including oxidation, reduction, and substitution.
Common Reagents and Conditions:
Major Products: These reactions yield ceftobiprole derivatives with modified functional groups.
Scientific Research Applications
Chemistry: Ceftobiprole serves as a valuable tool for studying antibiotic resistance mechanisms and drug design.
Biology: Researchers explore its interactions with PBPs and bacterial cell walls.
Industry: Pharmaceutical companies utilize ceftobiprole in drug development.
Comparison with Similar Compounds
Uniqueness: Ceftobiprole’s affinity for MRSA and activity against divergent mecA gene homologues set it apart.
Similar Compounds: While ceftobiprole stands out, other cephalosporins (e.g., ceftriaxone, cefepime) share structural similarities.
Properties
CAS No. |
376653-43-9 |
---|---|
Molecular Formula |
C26H26N8O11S2 |
Molecular Weight |
690.7 g/mol |
IUPAC Name |
(6R,7R)-7-[[(2E)-2-(5-amino-1,2,4-thiadiazol-3-yl)-2-hydroxyiminoacetyl]amino]-3-[(Z)-[1-[(3R)-1-[(5-methyl-2-oxo-1,3-dioxol-4-yl)methoxycarbonyl]pyrrolidin-3-yl]-2-oxopyrrolidin-3-ylidene]methyl]-8-oxo-5-thia-1-azabicyclo[4.2.0]oct-2-ene-2-carboxylic acid |
InChI |
InChI=1S/C26H26N8O11S2/c1-10-14(45-26(41)44-10)8-43-25(40)32-4-3-13(7-32)33-5-2-11(20(33)36)6-12-9-46-22-16(21(37)34(22)17(12)23(38)39)28-19(35)15(30-42)18-29-24(27)47-31-18/h6,13,16,22,42H,2-5,7-9H2,1H3,(H,28,35)(H,38,39)(H2,27,29,31)/b11-6-,30-15+/t13-,16-,22-/m1/s1 |
InChI Key |
HFTSMHTWUFCYMJ-ZOZZGPHGSA-N |
Isomeric SMILES |
CC1=C(OC(=O)O1)COC(=O)N2CC[C@H](C2)N3CC/C(=C/C4=C(N5[C@@H]([C@@H](C5=O)NC(=O)/C(=N/O)/C6=NSC(=N6)N)SC4)C(=O)O)/C3=O |
SMILES |
CC1=C(OC(=O)O1)COC(=O)N2CCC(C2)N3CCC(=CC4=C(N5C(C(C5=O)NC(=O)C(=NO)C6=NSC(=N6)N)SC4)C(=O)O)C3=O |
Canonical SMILES |
CC1=C(OC(=O)O1)COC(=O)N2CCC(C2)N3CCC(=CC4=C(N5C(C(C5=O)NC(=O)C(=NO)C6=NSC(=N6)N)SC4)C(=O)O)C3=O |
Origin of Product |
United States |
Retrosynthesis Analysis
AI-Powered Synthesis Planning: Our tool employs the Template_relevance Pistachio, Template_relevance Bkms_metabolic, Template_relevance Pistachio_ringbreaker, Template_relevance Reaxys, Template_relevance Reaxys_biocatalysis model, leveraging a vast database of chemical reactions to predict feasible synthetic routes.
One-Step Synthesis Focus: Specifically designed for one-step synthesis, it provides concise and direct routes for your target compounds, streamlining the synthesis process.
Accurate Predictions: Utilizing the extensive PISTACHIO, BKMS_METABOLIC, PISTACHIO_RINGBREAKER, REAXYS, REAXYS_BIOCATALYSIS database, our tool offers high-accuracy predictions, reflecting the latest in chemical research and data.
Strategy Settings
Precursor scoring | Relevance Heuristic |
---|---|
Min. plausibility | 0.01 |
Model | Template_relevance |
Template Set | Pistachio/Bkms_metabolic/Pistachio_ringbreaker/Reaxys/Reaxys_biocatalysis |
Top-N result to add to graph | 6 |
Feasible Synthetic Routes
Disclaimer and Information on In-Vitro Research Products
Please be aware that all articles and product information presented on BenchChem are intended solely for informational purposes. The products available for purchase on BenchChem are specifically designed for in-vitro studies, which are conducted outside of living organisms. In-vitro studies, derived from the Latin term "in glass," involve experiments performed in controlled laboratory settings using cells or tissues. It is important to note that these products are not categorized as medicines or drugs, and they have not received approval from the FDA for the prevention, treatment, or cure of any medical condition, ailment, or disease. We must emphasize that any form of bodily introduction of these products into humans or animals is strictly prohibited by law. It is essential to adhere to these guidelines to ensure compliance with legal and ethical standards in research and experimentation.