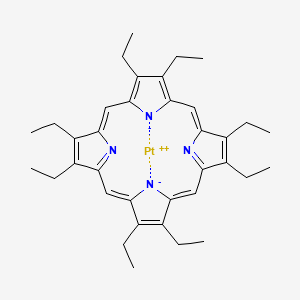
PtOEP
Overview
Description
PtOEP, also known as Platinum octaethylporphyrin, is an oxygen-quenchable luminescent dye . It is used as a fluorescent dye in the fabrication of polymer and silica-based oxygen sensors . It is also used as a red triplet emitting material with high external quantum yields for OLEDs .
Chemical Reactions Analysis
PtOEP is involved in a process called triplet-triplet annihilation upconversion (TTA-UC), which is a photochemical process where two lower energy photons are used to produce one photon of higher energy . This process is often performed by a two-component system, with PtOEP acting as a “triplet sensitizer” and another molecule acting as an "emitter" .
Scientific Research Applications
Optical Oxygen Sensors
PtOEP has found significant use in optical oxygen sensors due to its advantageous properties. Here’s why:
- Sensor Fabrication : Researchers have developed a low-cost and highly sensitive dissolved oxygen optical sensor using a membrane of polydimethylsiloxane (PDMS) doped with PtOEP. The PDMS matrix offers excellent mechanical and chemical properties, including high oxygen permeability and anti-biofouling characteristics .
- Sensitivity : PtOEP’s strong phosphorescence at room temperature, with a quantum yield close to 50%, makes it ideal for luminescence-based oxygen sensing. The sensor can detect low oxygen concentrations in both gaseous (0.5% to 20%) and liquid media (0.5 mg/L to 3.3 mg/L) at 1 atm and 25°C .
In Vivo Imaging Sensitizers
Beyond sensors, PtOEP has applications in in vivo imaging:
- Lanthanide Complexes : Researchers have employed lanthanide complexes of porphyrinoids (including PtOEP) inside nanomicelles and mesoporous silica nanoparticles for in vivo imaging. These complexes act as sensitizers in triplet-triplet annihilation upconversion (TTA-UC) processes, demonstrating their potential in cellular imaging .
Fluorescent Microspheres
PtOEP has been used in the synthesis of fluorescent microspheres:
- Monodispersed PS/PtOEP Microspheres : Researchers successfully synthesized monodispersed polystyrene (PS)/PtOEP microspheres via dispersion polymerization. These microspheres hold promise for various applications, including fluorescence-based sensing and imaging .
Ratiometric Oxygen Sensors
PtOEP contributes to ratiometric oxygen sensors:
- PtOEP-C6/Poly(St-TFEMA) Sensor : A ratiometric oxygen sensor was developed using PtOEP as the oxygen-dependent indicator and C6 as the oxygen-independent indicator. This sensor exhibits high sensitivity, strong anti-interference ability, and accurate detection capabilities .
Mechanism of Action
Target of Action
Platinum Octaethylporphyrin (PtOEP) is a type of metalloporphyrin that primarily targets oxygen molecules . It is used as a fluorescent dye in the fabrication of oxygen sensors and as a sensitizer in upconversion organogels .
Mode of Action
PtOEP interacts with its targets through a process known as phosphorescence. This process involves the absorption of light by the PtOEP molecule, which then transitions from a singlet state to a triplet state . The triplet state is longer-lived and allows for energy transfer to oxygen molecules . This energy transfer results in the quenching of the phosphorescence, which can be measured and correlated to the concentration of oxygen .
Biochemical Pathways
The primary biochemical pathway affected by PtOEP is the phosphorescence pathway. When PtOEP absorbs light, it transitions to an excited state. This excited state can then undergo intersystem crossing (ISC) to reach a triplet state . The triplet state of PtOEP can interact with oxygen molecules, leading to the quenching of phosphorescence .
Result of Action
The primary result of PtOEP’s action is the quenching of phosphorescence in the presence of oxygen. This quenching can be measured and used to determine the concentration of oxygen in a given environment . Additionally, PtOEP has been shown to have strong phosphorescence at room temperature with a quantum yield close to 50%, making it an effective sensor for oxygen .
Action Environment
The action of PtOEP is influenced by several environmental factors. For instance, the presence of oxygen in the environment leads to the quenching of PtOEP’s phosphorescence . Additionally, the physical form of PtOEP (e.g., thin film or nanoparticle) can influence its action and efficacy . Furthermore, PtOEP has been shown to have high oxygen sensitivity, strong anti-interference ability, and accurate detection capabilities, making it suitable for use in a variety of environments .
Safety and Hazards
Future Directions
PtOEP has potential applications in photobiocatalysis and in vivo molecular imaging and drug delivery . For example, PtOEP can be used in TTA-UC poly(styrene) nanoparticles for application in aqueous solutions . Also, the advantageous properties of PLGA can be combined with the unique optical properties of TTA-UC for the development of advanced nanocarriers for simultaneous in vivo molecular imaging and drug delivery .
properties
IUPAC Name |
2,3,7,8,12,13,17,18-octaethylporphyrin-22,24-diide;platinum(2+) | |
---|---|---|
Details | Computed by Lexichem TK 2.7.0 (PubChem release 2021.05.07) | |
Source | PubChem | |
URL | https://pubchem.ncbi.nlm.nih.gov | |
Description | Data deposited in or computed by PubChem | |
InChI |
InChI=1S/C36H44N4.Pt/c1-9-21-22(10-2)30-18-32-25(13-5)26(14-6)34(39-32)20-36-28(16-8)27(15-7)35(40-36)19-33-24(12-4)23(11-3)31(38-33)17-29(21)37-30;/h17-20H,9-16H2,1-8H3;/q-2;+2 | |
Details | Computed by InChI 1.0.6 (PubChem release 2021.05.07) | |
Source | PubChem | |
URL | https://pubchem.ncbi.nlm.nih.gov | |
Description | Data deposited in or computed by PubChem | |
InChI Key |
WAODGUVBNLMTSF-UHFFFAOYSA-N | |
Details | Computed by InChI 1.0.6 (PubChem release 2021.05.07) | |
Source | PubChem | |
URL | https://pubchem.ncbi.nlm.nih.gov | |
Description | Data deposited in or computed by PubChem | |
Canonical SMILES |
CCC1=C(C2=CC3=NC(=CC4=C(C(=C([N-]4)C=C5C(=C(C(=N5)C=C1[N-]2)CC)CC)CC)CC)C(=C3CC)CC)CC.[Pt+2] | |
Details | Computed by OEChem 2.3.0 (PubChem release 2021.05.07) | |
Source | PubChem | |
URL | https://pubchem.ncbi.nlm.nih.gov | |
Description | Data deposited in or computed by PubChem | |
Molecular Formula |
C36H44N4Pt | |
Details | Computed by PubChem 2.1 (PubChem release 2021.05.07) | |
Source | PubChem | |
URL | https://pubchem.ncbi.nlm.nih.gov | |
Description | Data deposited in or computed by PubChem | |
Molecular Weight |
727.8 g/mol | |
Details | Computed by PubChem 2.1 (PubChem release 2021.05.07) | |
Source | PubChem | |
URL | https://pubchem.ncbi.nlm.nih.gov | |
Description | Data deposited in or computed by PubChem | |
Product Name |
PtOEP |
Retrosynthesis Analysis
AI-Powered Synthesis Planning: Our tool employs the Template_relevance Pistachio, Template_relevance Bkms_metabolic, Template_relevance Pistachio_ringbreaker, Template_relevance Reaxys, Template_relevance Reaxys_biocatalysis model, leveraging a vast database of chemical reactions to predict feasible synthetic routes.
One-Step Synthesis Focus: Specifically designed for one-step synthesis, it provides concise and direct routes for your target compounds, streamlining the synthesis process.
Accurate Predictions: Utilizing the extensive PISTACHIO, BKMS_METABOLIC, PISTACHIO_RINGBREAKER, REAXYS, REAXYS_BIOCATALYSIS database, our tool offers high-accuracy predictions, reflecting the latest in chemical research and data.
Strategy Settings
Precursor scoring | Relevance Heuristic |
---|---|
Min. plausibility | 0.01 |
Model | Template_relevance |
Template Set | Pistachio/Bkms_metabolic/Pistachio_ringbreaker/Reaxys/Reaxys_biocatalysis |
Top-N result to add to graph | 6 |
Feasible Synthetic Routes
Q & A
Q1: What is the molecular formula and weight of PtOEP?
A1: PtOEP has the molecular formula C36H44N4Pt and a molecular weight of 707.85 g/mol.
Q2: What are the key spectroscopic features of PtOEP?
A: PtOEP displays strong absorption in the UV-vis region, with characteristic Soret and Q bands. It exhibits intense phosphorescence at room temperature, unlike many organic molecules, with a peak typically around 650 nm. [, , ]
Q3: Why is PtOEP's room-temperature phosphorescence significant?
A: This property, arising from the heavy atom effect of platinum, enables its use in various optical sensing applications, including oxygen sensing, where the long phosphorescence lifetime allows for sensitive detection of oxygen quenching. [, , , ]
Q4: Is PtOEP compatible with common polymer matrices for device fabrication?
A: Yes, PtOEP has been successfully incorporated into a range of polymer hosts, including polystyrene (PS), poly(methyl methacrylate) (PMMA), poly(9,9-dioctylfluorene) (PFO), and polydimethylsiloxane (PDMS), demonstrating its versatility for device fabrication. [, , , , , ]
Q5: Does PtOEP aggregate in these matrices, and how does this impact its properties?
A: The aggregation behavior of PtOEP is influenced by its concentration and the nature of the host matrix. At higher concentrations and in certain hosts, PtOEP tends to form aggregates, which can alter its photophysical properties, such as phosphorescence lifetime and quantum yield. [, , ]
Q6: How does temperature affect PtOEP's luminescence and energy transfer processes in solid films?
A: Lowering the temperature generally enhances both PtOEP phosphorescence and the efficiency of triplet-triplet annihilation (TTA) upconversion processes in PtOEP-containing composites. This is attributed to reduced non-radiative decay pathways and enhanced energy transfer at lower temperatures. [, ]
Q7: How stable is PtOEP under continuous photoexcitation, and what factors influence its photostability?
A: PtOEP exhibits good photostability, but prolonged exposure to UV irradiation, particularly in the presence of oxygen, can lead to degradation. Incorporation into protective matrices and the use of less energetic excitation wavelengths can improve its photostability. [, ]
Q8: What is triplet-triplet annihilation upconversion (TTA-UC), and how is PtOEP involved?
A: TTA-UC is a process where two low-energy triplet excitons combine to produce one higher-energy singlet exciton, leading to the emission of a higher-energy photon. PtOEP acts as an efficient sensitizer in TTA-UC systems, absorbing lower-energy photons and transferring the energy to an acceptor molecule, which then undergoes TTA to emit upconverted light. [, , , , ]
Q9: What factors influence the efficiency of TTA-UC in PtOEP-based systems?
A: Key factors include the choice of acceptor molecule, the concentration and distribution of PtOEP and the acceptor in the solid matrix, the efficiency of triplet energy transfer from PtOEP to the acceptor, and the presence of any quenching pathways that compete with TTA. [, , , , , ]
Q10: Can you provide an example of a successful TTA-UC system incorporating PtOEP?
A: A highly efficient solid-state TTA-UC system was achieved using PtOEP as the sensitizer and a bulky 9,10-diphenylanthracene (DPA) derivative as the emitter. The bulky substituents on DPA improved the dispersion of PtOEP within the emitter crystals, leading to a significant enhancement in the TTA-UC quantum yield. []
Q11: How is PtOEP employed in optical oxygen sensors?
A: PtOEP's phosphorescence is highly sensitive to the presence of oxygen. As oxygen concentration increases, the phosphorescence intensity and lifetime decrease proportionally due to collisional quenching. This phenomenon is the basis for PtOEP-based optical oxygen sensors. [, , , , , ]
Q12: What are the advantages of using PtOEP in optical oxygen sensors?
A: PtOEP offers several advantages, including high sensitivity to oxygen, a large Stokes shift, relatively long phosphorescence lifetime (allowing for time-resolved measurements), good photostability, and compatibility with various immobilization matrices. [, , , , , ]
Q13: Are there different methods to measure the oxygen-dependent phosphorescence of PtOEP in sensors?
A: Yes, both intensity-based and lifetime-based methods can be employed. Intensity-based sensing relies on measuring the decrease in phosphorescence intensity with increasing oxygen concentration, while lifetime-based sensing monitors the decrease in phosphorescence lifetime. Lifetime-based methods can be advantageous as they are less susceptible to fluctuations in excitation intensity or dye concentration. [, , ]
Q14: What is the role of the matrix material in PtOEP-based oxygen sensors?
A: The matrix material influences the sensor's performance by affecting the oxygen permeability, the homogeneity of PtOEP dispersion, and the long-term stability of the sensing film. [, , , ]
Disclaimer and Information on In-Vitro Research Products
Please be aware that all articles and product information presented on BenchChem are intended solely for informational purposes. The products available for purchase on BenchChem are specifically designed for in-vitro studies, which are conducted outside of living organisms. In-vitro studies, derived from the Latin term "in glass," involve experiments performed in controlled laboratory settings using cells or tissues. It is important to note that these products are not categorized as medicines or drugs, and they have not received approval from the FDA for the prevention, treatment, or cure of any medical condition, ailment, or disease. We must emphasize that any form of bodily introduction of these products into humans or animals is strictly prohibited by law. It is essential to adhere to these guidelines to ensure compliance with legal and ethical standards in research and experimentation.